Breast cancer related lymphedema—novel regenerative therapies: a narrative review
Introduction
Breast cancer is the most common cancer in women (1). The treatment of breast cancer often includes surgery in the axillary area, either sentinel lymph node (LN) biopsy or LN evacuation (2). The iatrogenic damage to the LNs and vessels and/or postoperative radiotherapy, chemotherapy, obesity and formation of seroma can cause a disruption of lymph flow causing lymphedema (3,4). The cumulative incidence of clinically manifested lymphedema after breast cancer treatment can be up to 41.1% (5,6). Chronic lymphedema first manifests with accumulation of interstitial fluid and pitting edema in the affected arm and is later accompanied with irreversible accumulation of fibro-adipose tissue and non-pitting edema (7). The excess fluid and tissue cause pain and decrease the function of the affected arm (8).
Despite multiple attempts the pathophysiology of lymphedema is still not fully understood. Fibrosis and scarring are known to be the key inhibitors of lymphatic regeneration (9,10). Transforming growth factor β1 (TGF-β1) and interleukin 10 (IL-10) are factors in chronic inflammation and formation of fibrosis, therefore they are highly interesting to lymphedema researchers (11). An immunological shift towards a Th2 cytokine response can potentially induce lymphedema (12-14). Tumor necrosis factor α (TNF-α) and vascular endothelial growth factor C (VEGF-C) have been shown to have pro-lymphangiogenic properties. TNF-α, a pro-inflammatory cytokine, stimulate the production of VEGF-C by fibroblasts (15,16). Lymphatic stasis is also a known factor behind chronic inflammation and tissue fibrosis, worsening lymphatic function (9,12). In a mouse model the gene expression profiles are similar in lymphedema and inflammation (14,17).
A definitive cure for secondary lymphedema is yet to be found. The conservative treatment options for lymphedema do not affect the root cause of the disease—lymphatic injury. Compression garments and manual lymphatic drainage aim to prevent fluid accumulation (18-20). More recently, surgical treatment options have been developed, including autologous lymph node transfer (ALNT) and lymphatic venous anastomosis (LVA). These procedures require specialized microsurgical techniques and are not available at every hospital. Additional liposuction (LIPO) is also needed for some patients to address the excess adipose tissue and not all patients benefit from these procedures (19,21-23). Therefore, developing effective, less invasive treatments, that treat the root cause of the disease or improve the existing treatment methods, is important.
This review focuses on the novel therapeutic approaches in the treatment of lymphedema, including bioengineering, growth factor-based therapies, immunomodulation and stem cell therapy. Previous reviews have focused mainly on pharmacological treatments (24). We review the regenerative options for optimizing the surgical treatment of lymphedema. Both preclinical studies and clinical trials are summarized. We present this article in accordance with the Narrative Review reporting checklist (available at https://abs.amegroups.com/article/view/10.21037/abs-22-38/rc).
Methods
We searched the PubMed Medline database for articles written in English during the years 2000–2022. Included studies were all peer-reviewed articles, original articles, case reports and literature reviews (Table 1).
Table 1
Items | Specification |
---|---|
Date of search | July 2022, search for updated data August 2023 |
Databases and other sources searched | PubMed |
Search terms used | “Lymphedema” [MeSH] AND “Regenerative therapies” [MeSH] |
“Lymph node transfer” [MeSH] AND “Regenerative therapies” [MeSH] | |
“Lymphedema” [MeSH] AND “Growth factor therapy” [MeSH] | |
“Lymph node transfer” [MeSH] AND “Growth factor therapy” [MeSH] | |
Timeframe | Jan 01, 2000 to Dec 31, 2022 |
Inclusion criteria | Research articles, case reports and reviews in English |
Exclusion criteria | Papers with low reliability |
Selection process | All authors conducted the selection and approved the literature selection |
Novel therapies
Bioengineering
In recent years, tissue engineering has had promising results in the field of immunology in developing artificial lymphatic networks and LNs. These innovations offer potential for correcting lymphatic vascular network defects, addressing immunodeficiency, autoimmune diseases, infections, and malignancies (25,26). For example, lymph vessel grafts could be used to surpass iatrogenic defects or congenital blockages of the lymphatic circulation (27).
The process of bioengineering blood vessels is already well known. Although it can partly be utilized for the lymphatic vasculature, lymph vessels possess certain unique characteristics (28). Lymphatic vessel is defined by both low pressure and lack of pressure pulses, illustrated by the lower strength and elasticity. Collecting lymphatics feature unidirectional flow maintenance via valves (26,27). Furthermore, the microarchitecture of the lymphatic microcapillaries differs from that of arterioles and venules (28). Other relevant features are biocompatibility, ability for biofunctionalization and endothelialization, adequate mechanical resilience, flexibility, resistance to fatigue and kinking and, furthermore, suture retention. Ensuring patient safety necessitates noncarcinogenic, nontoxic and nonallergenic grafts (27).
Three-dimensional (3D) matrices and scaffolds
In general, most approaches for developing tissue-engineered lymphatic grafts utilize biomaterial-based 3D matrices, scaffolds (25,27). These structures facilitate interaction between the scaffold, cells, growth factors, and cytokines, fostering an environment for lymphatic endothelial cell (LEC) growth and regeneration (25). The challenge lies in achieving self-organization of endothelial cells into functional networks in vivo, particularly for creating artificial replacements with intraluminal valves for collecting lymphatic vessels (27).
The scaffolds can be natural, manufactured from modified natural-origin proteins and decellularized, or produced using synthetic polymers (25,27). Synthetic scaffolds can be either biodegradable or nonbiodegradable (25,27).
Research has demonstrated that in vitro lymphatic capillary formation is possible by embedding LECs in a 3D matrix and applying an interstitial flow to enhance proliferation. These cultured grafts could be implanted in vivo to reconstruct the lymphatic network. In a study on rats using fibrin hydrogel containing LECs, these bioengineered lymph capillaries achieved lymph perfusion and connection with host vessels (28). Another study (29) on rats using a decellularized dermal matrix showed rapid ingrowth of lymphatic (and blood) vessels into the matrix, with only minor inflammatory response due to the decellularization process. These findings suggest potential for functional reconstruction. However, recreation of lymphatic networks with adequate support structures (e.g., valves and smooth muscle coverage) necessary for unidirectional lymph flow is significantly more complex (30).
VEGF-C
In terms of lymphatic vascular network replacement by tissue engineering, lymphangiogenesis-inducing growth factors have been utilized with promising results in various animal models (30,31). Of all the candidates, VEGF-C has an established position in lymphedema research. It has been demonstrated to be essential in adult lymphatic vessel regeneration and is widely considered as the primary growth factor of choice for therapeutic applications involving LECs (32,33). VEGF-C therapy has been demonstrated to induce the growth of capillary lymph vessels, which are thought to stabilize into true collecting lymph vessels via an intrinsic differentiation and maturation program (32). Consequently, the use of lymphangiogenic growth factors possesses significant potential as an adjunct to engineered biomaterials. They could be administered locally or systemically to induce lymph vessel formation and to provide greater biocompatibility for the various biomaterials (27).
Nanofibrillar collagen scaffold
BioBridgeTM (Fibralign Corporation, Union City, CA, USA) is a nanofibrillar collagen scaffold, which can enhance lymphangiogenesis by providing the regenerating lymph vessels a mechanical structure to follow. It presents potential applications in existing surgical procedures for lymphedema, such as ALNT, LVA and LIPO (34).
BioBridgeTM treatment protocol has been provided and demonstrated both in a preclinical and clinical setting in a study by Nguyen et al. (29) The study was made with a rat model, supplemented by human case reports, and demonstrated the capacity of BioBridgeTM to enhance the results of ALNT and LVA and also prevent lymphedema. The design involved three groups: a prevention group that underwent lymphadenectomy and received simultaneous BioBridgeTM treatment; a treatment group that, after developing lymphedema following a lymphadenectomy, received the combination of BioBridgeTM treatment with seeded adipose-derived stem cells (ADSC); a control group with no treatment after lymphadenectomy. The rats in the treatment group exhibited a greater reduction in the volume of the affected limb and backflow in magnetic resonance imaging (MRI) lymphangiography and an increase in the number of collecting lymph vessels in MRI lymphangiography compared to the control group. Similarly, the prevention group showed a lower incidence of lymphedema. Similar promising results were seen in human case reports (29).
The BioBridgeTM treatment has also been examined in a porcine model of acquired lymphedema model. The study compared different treatment approaches, including BioBridgeTM alone, BioBridgeTM with ALNT, standalone surgery, and BioBridgeTM combined with exogenous VEFG-C-growth factor (35). The number of collecting lymph vessels increased significantly in all the porcines treated with BioBridgeTM. However, a significant improvement in lymphatic drainage, estimated using bioimpedance as a marker of extracellular fluid accumulation, was seen only in the experimental treatment group (35). These results collectively emphasize the beneficial impact of BioBridgeTM.
Nanofibrillar collagen scaffold—BioBridgeTM—clinical studies
In addition, Deptula et al. reported a new triple therapy algorithm for optimizing the treatment protocol of late stage (II–III) lymphedema for each patient, treated individually with the BioBridgeTM (36). The study was retrospective, and patients were offered different treatment protocols according to stage of lymphedema and lymphatic mapping. The patients with mostly fibroadipose component of lymphedema (stage III) first underwent a debulking LIPO. After a year, lymphatic mapping was performed in order to determine the treatment protocol. For the patients with blocked distal superficial lymphatics, LVA was offered, and for those with a history of a cellulitis, also ALNT. Patients with no targetable blocked distal superficial lymphatics were offered ALNT. In contrast, patients with both fibroadipose and fluid component of lymphedema (late stage II) were offered the combination of a LIPO and ALNT or LVA. Additional LIPO was performed when needed, while in the case of excess fluid volume, BioBridgeTM scaffolds placed percutaneously into the subcutaneous layer were utilized. The 14 treated patients had a 28.5% reduction in median excess volume (29% to 0.5%) during the first 14.4 months after the first stage surgery and furthermore improved an additional 1.5% after the triple therapy treatment with BioBridgeTM after 24.6 months of follow-up. These results were statistically significant, P<0.01 (36). Dr. Nguyen’s group have also reported a retrospective cohort study in stage I–III patients with similar results regarding volume reduction, new lymphatic collectors and reduced dermal backflow. These studies are limited by the retrospective nature and the small number of patients with varying lymphedema stages (37).
Interestingly, the underlying mechanism behind the benefit from the BioBridgeTM treatment in LVA patients is under discussion. It can be speculated that the improvement may not be only due to the formation of new collecting lymph vessels, but also due to the scaffolds creating a path of lesser resistance, hence improving the lymph flow, as discussed by Shuck et al. (38).
Furthermore, the BioBridgeTM scaffolds have also been shown to enhance the function of endothelial cells by increasing the cell survival and inhibiting inflammation (34,39). This could affect the results since inflammatory response is known to have a role in the formation of lymphedema (40,41).
Replacement of LNs
Similar to bioengineered lymph vessels, creating artificial LNs often involves biomaterial-based 3D scaffolds resembling the extracellular matrix seen in animal tissue. These scaffolds are manufactured from modified natural origin or synthetic polymers. They can be cell-free and contain, for example, a mixture of different cytokines and growth factors. After implantation, the scaffold forms a foundation for an artificial organ, aiming to attract progenitor cells for LN development, ultimately simulating a natural LN (26,30).
In a mouse model, collagen gel scaffolds seeded with dendritic cells and lymphotoxin-α (LT-α)-expressing thymic stromal cells successfully generated functional artificial lymphoid organs. These organs exhibited cellular reorganization, lymphocyte chemoattraction, clustering, high endothelial venule ingrowth, and antigen-specific antibody production (30). Another promising, but more complex strategy involves using patient-derived induced pluripotent stem cells (iPSC) to replace the scaffolds and establish primary cultures for LN formation, which can then be implanted. Combining these methods could optimize the results (26).
The use of artificial LNs could reduce donor-site morbidity and improve recovery after ALNT, potentially substituting axillary or inguinal LNs removed due to malignancies. Bridging the defect between the LN and the lymph vessels with bioengineered lymph vessel grafts is an interesting possibility in the future (28,30,39,42).
These strategies demand in-depth understanding of lymphoid organogenesis and immunological signaling. They offer results exceeding the potential of natural lymphoid organs and immune cells. Artificial lymphoid organs may be of great benefit in treating autoimmune diseases, immunodeficiencies and cancer, but their role in the surgical treatment of chronic lymphedema remains still to be determined (26). Presently, these artificial organs do not increase lymph flow, but they mark progress in the alternatives for the treatment of lymphedema (28,30,39,42).
Growth factor-based treatment
VEGF-C
VEGF-C has been shown to ameliorate lymphedema, induce lymphangiogenesis and enhance the survival, function and lymphatic network integration of the transplanted LNs (30). The use of VEGF-C treatment aims at restoring the normal anatomy and function of both collecting and capillary lymphatics. As a result, lymph fluid drainage increases, edema alleviates, mechanical tension decreases and inflammation mitigates. Also, the density of lymphatic capillaries is increased locally, resulting in accelerated immune cell trafficking to the draining LNs. This enhances the protective immune response around the surgical wound and availability of cytokines and growth factors (42).
VEGF-C can be delivered as a recombinant protein, viral vector or naked plasmid. It can be administered directly or released on-demand from bioengineered matrices or biodegradable microparticles (30). Therapy with recombinant VEGF-C-expressing adeno-associated virus is considered as the most efficient method of delivering genes in vivo and it has been tested using various experimental animal models (31,33,42-47).
VEGF-C—clinical studies Lymfactin®
Lymfactin is an adenovirus type 5-based gene therapy (Lymfactin®), involving the expression of human VEGF-C, and it has been used in combination with ALNT (Figure 1). The medicinal product Lymfactin® was provided by Herantis Pharma. A phase I study by Hartiala et al. combined Lymfactin® therapy with ALNT in order to treat upper limb lymphedema (48). Fifteen patients were recruited between June 2016 and February 2018, three receiving a lower dose [1×1010 viral particles (vp)] and 12 patients a higher dose (1×1011 vp) of Lymfactin®, respectively. The results demonstrated Lymfactin to be safe and well-tolerated with no dose-limiting toxicities (48). In the higher dose group, an average reduction of 46% in the excess upper limb volume after a 12-month follow-up and the transport index was improved in 7/12 patients (49). At baseline, before the treatment, removal of compression garments for 7 consecutive days resulted in a significant increase in upper limb swelling (105.7±161.0 mL, P<0.05). However, at 12 months postoperatively, removal of the garments resulted in a lesser and statistically not significant increase in the swelling (84.4±143.0 mL, P>0.05). Lymphedema Quality of Life Inventory (LyQoLI) questionnaire showed a significant and sustained improvement of quality of life (QOL) (49).
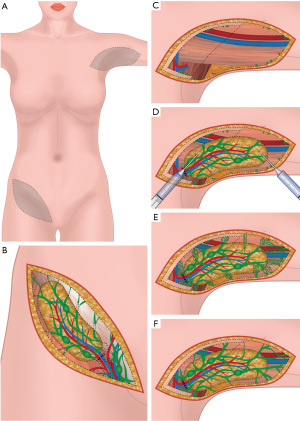
Consequently, a double-blind, randomized multicenter phase II study with Lymfactin® [5 centers: Finland (Helsinki, Turku, Tampere) and Sweden (Stockholm, Uppsala)] has been conducted (clinical trial registration number NCT03658967). The results regarding the therapeutic efficiency, safety and tolerability of Lymfactin® in 39 patients with BCRL are ready to be published. Volumetry, LyQoLI and lymphoscintigraphy showed a positive effect of an ALNT in both groups compared to the baseline, but without differences between the groups at 12 months. Noteworthy, there was a significantly greater improvement in the bioimpedance ratio, which measures the fluid component of lymphedema, in the Lymfactin® group compared to placebo group (P<0.05). No differences in adverse events were detected between the groups. These results indicate Lymfactin® treatment to be beneficial. Limitations of the study include the varying lymphedema stages of the patients. As Lymfactin® targets only the fluid component of lymphedema, in patients with late-stage lymphedema the treatment can not result in total reduction of the excess volume (48).
Hepatocyte growth factor (HGF)
HGF has also been investigated regarding its role in lymphangiogenesis (50). HGF is now known to be able to regulate tissue and organ regeneration and modulate cell morphology. It can also stimulate cell motility and migration, and regulate cell growth and death (51). HGF is highly interesting growth factor in the clinical setting due to the diverse potential in prognostic and therapeutic implications.
Kajiya et al. (51) found that LECs display elevated levels of HGF receptors compared to blood vascular cells. While the expression of HGF receptors is limited in normal lymphatic vessels, it increases in regenerating endothelium during tissue repair and in activated vessels during skin inflammation. Treating LECs with HGF boosts cell growth, migration, and tube formation. This highlights HGF’s importance in lymphatic vessel growth and proposes HGF receptor as a potential target for managing irregular lymphatic growth (51).
Saito et al. (52) examined the therapeutic potential of HGF in treating lymphedema. The researchers demonstrated that HGF treatment promoted cell growth, migration, and signaling pathways in LECs. Gene transfer of HGF in a rat model of lymphedema reduced swelling and increased expression of lymphatic markers. These findings highlight HGF’s potential for enhancing lymphangiogenesis and treating lymphedema. However, no further studies on the exploitation of HGF have followed probably owing to the multiple other effects of HGF which makes it inspecific for lymphedema treatment (52).
Immunomodulation
TGF-β1
The inhibition of lymphangiogenesis-inhibiting signals might offer a worthy alternative for VEGF-C and other growth factors, particularly due to concerns about potential contributions to tumor growth or metastasis (33,53). Despite the normal or increased expression of VEGF-C or other lymphangiogenic cytokines in certain pathological circumstances, lymphatic function can be impaired based on multiple studies (12-14). Therefore, it has been suggested that other physiological mechanisms could either directly or indirectly inhibit lymphangiogenesis (30). TGF-β1, a potent anti-lymphangiogenic agent, is noteworthy. It diminishes LEC proliferation and migration, disrupts lymphatic tubule formation and downregulates lymphatic-specific gene expression (33). TGF-β1 inhibition has been shown to enhance lymphatic repair during wound healing and synergistically amplify the lymphangiogenic effect of VEGF-C (30,53). Consequently, TGF-β1 inhibition could promote lymphangiogenesis and present an alternative to VEGF-C and other growth factors in clinical situations (30,33,54).
TGF-β1 also functions as an anti-inflammatory cytokine, regulating tissue fibrosis and scarring in the later stages of wound healing, thus linking lymphangiogenesis with inflammatory pathways. IL-10, another anti-inflammatory cytokine, guards against TGF-β1-induced fibrosis (40). Elevated IL-10 levels have been discovered after ALNT in humans and it could influence the effects of ALNT (53). An additional example how inflammatory pathway can intrude with VEGF pathway in order to induce lymph vessel growth is TNF-α, which can stimulate lymphangiogenesis directly via tumor necrosis factor receptor 1 or indirectly by activating inflammatory macrophages that produce VEGF-C (47). Furthermore, interferon gamma (INF-γ) can inhibit lymphangiogenesis irrespective of VEGF-C (and -A) (29). Intriguingly, the anti-lymphangiogenic effect of both TGF-β1 and INF-γ are related to T-cell inflammation, thus establishing a connection between lymphangiogenesis and inflammation (30).
Th2 inhibition
Lymphedema has been associated with a fibrotic Th2 type inflammatory response orchestrated by Th2 type CD4 helper T cells and macrophages (12,13). It has been suggested that macrophages have an anti-fibrotic role in lymphedema and regulate Th2 differentiation either directly or indirectly (55). Notably, recent research by Ghanta et al. provided evidence that lymphedema-associated macrophages are a substantial source of VEGF-C and that impaired macrophage response after lymphatic injury leads to compromised lymphatic function (55). In addressing this intricate interplay, neutralizing antibody inhibition of Th2 differentiation through interleukin 4 (IL-4) or interleukin 13 (IL-13) emerges as a possibility. These cytokines are necessary for differentiation of naïve CD4+ cells to the Th2 lineage. In a mouse tail model, inhibiting Th2 differentiation has been effective in both treatment and prevention of lymphedema. Mice treated in this manner have decreased fibroadipose tissue deposition, ameliorated inflammation, improved lymphatic collecting vessel pumping capacity, decreased lymphatic leakiness, and overall improved lymphatic function (13,14). By targeting the Th2 inflammatory response, this approach holds potential for addressing the complex mechanisms underpinning lymphedema progression.
Th2 inhibition—clinical studies
An experimental drug, QBX258, was studied by Mehrara et al. (56) in a phase I, open-label trial. It consists of two humanized monoclonal antibodies that inhibit IL-4 and IL-13. Patients with BCRL were treated once a month with an intravenous infusion of QBX258 for 4 months. Outcomes were analysed both immediately and 4 months after the withdrawal of the treatment. QBX258 treatment was demonstrated to be safe, and most adverse events were minor and self-limited. Treatment with QBX258 improved QOL measurements and alleviated both skin stiffness and histologic changes in the lymphedematous arm. However, no significant improvements in the arm volumes or bioimpedances were found. Notably, no randomized study has yet been conducted (56).
Leukotriene B4 (LTB4) and ketoprofen
Also worth mentioning, previous research has demonstrated that histopathology in experimental lymphedema can be reversed with ketoprofen, a nonsteroidal anti-inflammatory drug (NSAID) (57). Inhibition of 5-lipoxygenase metabolite, LTB4 is thought to be the reason for this effect. Low LTB4 concentrations have been shown to promote lymphangiogenesis both in in vitro and in vivo experimental animal models, whereas lymphatic growth and function are impeded at high concentrations. Interestingly, LTB4 concentration increase has been seen in both experimental animal model and lymphedema patients. It is speculated that during the initial wound-healing period, LTB4 produced at low concentrations has an important function in promoting angiogenesis/lymphangiogenesis. A shift from initial lymphangiogenesis-stimulating into anti-lymphangiogenic effect is seen when increase in LTB4 concentration occurs. This is why LTB4 is a promising drug target in the treatment of acquired lymphedema (57).
LTB4 and Ketoprofen—clinical studies
The beneficial effects of ketoprofen in the treatment of lymphedema have been reported by Rockson et al. (58). The study hypothesis was first tested in an open-label exploratory trial, followed by a placebo-controlled trial of 34 patients. No serious adverse events were detected. The patients treated with ketoprofen had a reduction in skin thickness, improvement in histopathology and reduction in plasma granulocyte-colony stimulating factor (G-CSF) expression when compared to the placebo group. However, arm volumes and bioimpedance results were similar between the groups (58). While treatment with ketoprofen in this study was reported to be safe with no serious adverse events, it is important to note that prolonged NSAID use is limited due to known toxicities [Food and Drug Administration (FDA), 2021; U.S. Prescribing information, 2021a].
Another anti-inflammatory drug treatment with acebilustat might be one of future possibilities (59). Acebilustat inhibits leukotriene A4 (LTA4) hydrolase and modulates LTB4. Rockson et al. has just launched a clinical phase II study comparing acebilustat to placebo (ClinicalTrials.govNCT05203835).
Stem cell therapy—preclinical models
In recent years, research has shown that the administration of ADSCs promotes lymphatic vascular network formation and alleviates lymphedema in rodent models of secondary lymphedema (60-62). In a study by Huang et al. (39), ADSCs acquired a lymphatic phenotype due to the sustained release of VEGF-C. However, several studies have concluded that ADSCs do not differentiate into lymphatic vessel cells, but rather release VEGF-C, which strongly promotes lymphangiogenesis (60,61,63-65). Using an x-irradiated mouse model of secondary lymphedema, Yoshida et al. reported an increased number of lymphatic vessels, increase in both VEGF-C and VEGFR-3 expression, and restoration of lymphatic function in a transplanted ADSC-count-dependent manner (66). In contrast, Takeda et al. reported that culturing human dermal LECs resulted in better cell proliferation, migration, and tube formation in ADSC-conditioned endothelial basal medium compared to basal medium supplemented with VEGF-C alone (67). Ogino et al. found that ADSC transplantation accelerated LEC proliferation, increased lymphatic vessel numbers, and mitigated fibrosis of the surrounding interstitial tissue (68). Therefore, it has been suggested that ADSCs secrete not only VEGF-C, but also other lymphangiogenic growth factors with synergistic effects, although the interplay between these growth factors on lymphangiogenesis remain to be elucidated (69).
Stem cell therapy—clinical studies
Fat can be used as a whole fat graft, i.e., no separation of cells is performed. However, for many regenerative purposes, the stromal vascular fraction (SVF) or ADSCs are isolated and used alone or in combination with fat grafting. There are some preliminary studies exploiting fat grafting or ADSCs either alone or in combination with ALNT (70). Maruccia et al. (70) compared ALNT to ALNT with fat grafting of the scar. ALNT with scar release and subcutaneous fat grafting at the wrist level was performed, without any scar removal to the axillary area. Their results showed a higher circumference reduction rate in ALNT + fat graft group (n=21) when compared to the ALNT group (n=18) (70). Toyserkani et al. have published several articles on combined fat grafting and ADSC treatment (71-75). In their first case report, a decrease in volume and increase in patient reported outcomes was observed. In their pilot study of 10 patients, a single injection of ADSCs alleviated lymphedema, based on patient-reported outcome measures, and no serious adverse events occurred (71). Five patients were able to reduce their use of conservative management. However, lymphoscintigraphic evaluation showed no improvement after ADSC treatment and no change in the excess arm volume was observed (71,72,75,76). Interestingly, there is another ongoing clinical trial (NCT02981485) regarding fat grafting in the treatment of lymphedema in China, but the records have not been updated (77).
Conclusions
Surgical procedures, such as ALNT and LVA, have yielded positive, but varying results in the treatment of lymphedema. LIPO is an efficient debulking method at late stages of lymphedema. The experimental studies have combined these surgical treatments with the induction of lymphangiogenesis by lymphatic scaffolds or growth factors (BioBridgeTM, Lymfactin®). Both methods have obtained promising results in animal models. However, in the human studies BioBridgeTM has not yet advanced to randomized prospective studies to prove efficacy. Lymfactin® was first investigated in a prospective phase I trial with promising results on both volume reduction, lymphoscintigraphy and QOL compared to baseline. Further, it was investigated in a randomized prospective phase II trial and showed positive effects in favor of the Lymfactin® group only for the tissue dielectric constant (TDC) ratio, not the above-mentioned primary outcome measures. There have also been concerns regarding the potential of VEGF-C in promoting growth of possible dormant tumor cells or metastasis. VEGF-C is naturally expressed by the LNs. Although VEGF-C expression is associated with a poor prognosis and increased metastasis risk in some cancer types, for breast cancer, the data is controversial and in some studies VEGF-C has even been associated with a better survival rate (78-80). In the Lymfactin® trial, the drug was administered on the operating table, ex vivo to minimize distant effects.
Studies targeting the inflammatory component of lymphedema (Th2 inhibition, LTB4 inhibition) have also shown positive effects in experimental models. In clinical studies they have shown reduction in skin thickness, but not a reduction in the excess volume of the arm compared to baseline.
Therapies that include ADSCs are still scarce, but based on preclinical data, they are likely to affect in two ways: by promoting lymphangiogenesis and also by modulating inflammation through secretion of IL-10 (81,82). ADSCs potentially can also modulate the fibrotic component of lymphedema, since they are used in hypertrophic scar treatment. However, the clinical studies are limited and the results less impressive than the preclinical studies.
Although considerable progress in lymphedema research has been made, the clinical trials have not resulted in a major breakthrough (Table 2). In experimental models, the defect in the lymphatic system and the following edema are very homogenous as opposed to patients. In the human patient, the pathophysiology of lymphedema after surgery and radiation includes fibrosis, inflammation, an extensive defect in the lymphatic system followed by lack of functionality in the lymphatics and at late stages, the accumulation of adipose tissue. The radiation induced scarring in the axilla is often extensive. As the problem is multifactorial, a multimodality approach seems to be the most feasible solution. A combination treatment with modulation of immune responses, induction of lymphangiogenesis combined with scar release and, also reconstructive surgery, would address several pathophysiology targets at once. ADSC treatment seems to also be a promising option. Clinical studies thus far are limited by the small number of patients and the comparison of studies is difficult because of the varying treatment, measurement, and follow-up protocols.
Table 2
Clinical study | Study subjects | Study setting | Measurements | Follow-up | Outcomes | Limitations |
---|---|---|---|---|---|---|
BioBridgeTM, nanofibrillar collagen scaffold, Nguyen et al. 2022 | (Preclinical) Rat model + (clinical) 2 human cases | Prevention group: BioBridgeTM with lymphadenectomy; Treatment group: lymphedema patients receiving BioBridgeTM with ADSCs; control group: no treatment after lymphadenectomy | Volumetry, MRI lymphangiography (number of collecting lymph vessels), incidence of lymphedema between prevention and control group | 4 months | A greater reduction in the volume of the affected limb; backflow and an increase in the number of collecting lymph vessels in MRI lymphangiography were observed in the treatment group compared to the control group, both in rats and humans | Only 2 human patients in clinical setting, short follow-up period |
BioBridgeTM + triple therapy algorithm, nanofibrillar collagen scaffold, Deptula et al. 2022 | 14 patients | Triple therapy algorithm for optimizing the treatment protocol of late stage (II–III) lymphedema treated individually (ALNT and/or LVA and/or BioBridgeTM and/or LIPO) | Lymphatic mapping, Volumetry | 24.6 months | Statistically significant reduction of volume of the affected arm in patients receiving BioBridgeTM | Retrospective, small number of patients |
BioBridgeTM, nanofibrillar collagen scaffold, Nguyen et al. 2021 | 29 patients (18 treatment, 11 control) | ALNT and/or LVA with or without delayed implantation of nanofibrillar collagen scaffold (BioBridgeTM) | Volumetry, Indocyanine green lymphatic mapping | 29 months | Volume reduction, new lymphatic collectors and reduced dermal backflow were seen in patients treated with BioBridgeTM | Retrospective, small number of patients |
Lymfactin®, adenovirus type 5-based gene therapy, VEGF-C, phase I, Hartiala et al. 2020 | 15 patients | Lymfactin® treatment combined with ALNT with or without breast reconstruction | Safety, adverse events and toxicities | 12 months | Lymfactin® is safe and well-tolerated with no dose-limiting toxicities | Short follow-up, small number of patients |
Lymfactin®, adenovirus type 5-based gene therapy, VEGF-C, phase II, Leppäpuska et al. 2022 | 15 patients | Lymfactin® treatment combined with ALNT with or without breast reconstruction | Volumetry with and without compression garment use, lymphoscintigraphy (TI), LyQoLI | 24 months | Significant reduction in excess volume at 12 months, TI reduced in 11/12 patients at 12 months, LyQoLI also showed a significant positive effect | Small number of patients, no control group |
Lymfactin®, adenovirus type 5-based gene therapy, VEGF-C, phase II, Rannikko et al. (in writing) | 39 patients (19 Lymfactin, 20 placebo) | A double-blind, randomized, placebo-controlled multicenter phase II study with Lymfactin® treatment combined with ALNT with or without breast reconstruction | Volumetry, LyQoLI, Tissue bioimpedance (TDC, Delfin), Lymphoscintigraphy (TI) | 12 months | Volumetry, LyQoLI and TI showed a positive effect in both groups compared to the baseline, but without differences between the groups at 12 months. TDC reduction was significantly greater in the Lymfactin® group compared to placebo group. | Small number of patients |
Experimental drug (QBX258), Inhibition of Th2 type expression of cytokines, phase I, Mehrara et al. 2021 | 9 patients | Phase I, open-label trial utilizing QBX258, an experimental drug consisting of two humanized monoclonal antibodies that inhibit IL-4 (VAK296) and IL-13 (QAX576) | Adverse events, volumetry, QOL, skin tonometry, bioimpedance (TDC) and expression of cytokines, 5 mm skin biopsies from the normal and lymphedematous limbs before and after treatment | 4 months of treatment + 4 months after the treatment | QBX258 is safe, and most adverse events were minor and self-limited. Improved QOL measurements and alleviated both skin stiffness and histologic changes. Significantly decreased keratinocyte hyperplasia, mast cell infiltration, and the expression of Th2 inducing cytokines in the skin. | Short follow-up time, not randomized |
Ketoprofen, open-label exploratory trial + double-blind, placebo-controlled trial, Rockson et al. 2018 | (Exploratory) 21 patients + (placebo-controlled) 34 patients: 16 treatment, 18 placebo | Patients with either primary or secondary lymphedema received ketoprofen 75 mg by mouth 3 times daily for 4 months | Changes in histopathology, skin thickness, volumetry, and tissue bioimpedance (TDC); systemic inflammatory mediators | 4 months | Reduction in skin thickness, improvement in histopathology and reduction in plasma granulocyte (G-CSF) expression were observed when compared to the placebo group. However, volumetry and bioimpedance results were similar between the groups | Short follow-up time, small number of patients |
Stem cell therapy, ADSCs, preliminary study, Maruccia et al. 2019 | 41 patients (21 fat grafting of the scar + ALNT; 18 ALNT alone) | ALNT with only scar release and subcutaneous fat grafting was performed at the wrist level, without any scar removal | Circumference of the arm, lymphoscintigraphy, QOL | Mean 29–32 months | A higher circumference reduction rate in ALNT + fat graft group, when compared to the ALNT group | Small number of patients, proper scar release was not performed and fat grafting and ADSC injection was performed subcutaneously without an incision into the axilla |
Stem cell therapy, fat grafting with ADSCs, case reports + pilot study, Toyserkani et al. 2019 | (Case report) 1 patient + (pilot study) 10 patients | Fat grafting and ADSC injection in treatment of lymphedema | Patient reported outcomes, volumetry, lymphoscintigraphy, use of compression garments | Case report: 4 months; pilot study: 12 months | Alleviated patient reported outcomes and lesser symptoms | Short follow-up time, small number of patients |
MRI, magnetic resonance imaging; ADSC, adipose-derived stem cell; ALNT, autologous lymph node transfer; LIPO, liposuction; LVA, lymphatic venous anastomosis; LyQoLI, Lymphedema Quality of Life Inventory; VEGF-C, vascular endothelial growth factor C; TI, transport index; TDC, tissue dielectric constant; IL, interleukin; QOL, quality of life; G-CSF, granulocyte-colony stimulating factor.
There are promising novel therapies arising for BRCL. However, clinical studies are still scarce and experience from the above-mentioned clinical studies should be exploited to improve the quality, methodology and patient selection for future clinical trials.
Acknowledgments
Funding: None.
Footnote
Provenance and Peer Review: This article was commissioned by the Guest Editors (Summer Hanson and David Chang) for the series “Breast Cancer-related Lymphedema” published in Annals of Breast Surgery. The article has undergone external peer review.
Reporting Checklist: The authors have completed the Narrative Review reporting checklist. Available at https://abs.amegroups.com/article/view/10.21037/abs-22-38/rc
Peer Review File: Available at https://abs.amegroups.com/article/view/10.21037/abs-22-38/prf
Conflicts of Interest: All authors have completed the ICMJE uniform disclosure form (available at https://abs.amegroups.com/article/view/10.21037/abs-22-38/coif). The series “Breast Cancer-related Lymphedema” was commissioned by the editorial office without any funding or sponsorship. P.H. has received honoraria for participating in advisory boards of Herantis Pharma Plc. (Espoo, Finland). The authors have no other conflicts of interest to declare.
Ethical Statement: The authors are accountable for all aspects of the work in ensuring that questions related to the accuracy or integrity of any part of the work are appropriately investigated and resolved.
Open Access Statement: This is an Open Access article distributed in accordance with the Creative Commons Attribution-NonCommercial-NoDerivs 4.0 International License (CC BY-NC-ND 4.0), which permits the non-commercial replication and distribution of the article with the strict proviso that no changes or edits are made and the original work is properly cited (including links to both the formal publication through the relevant DOI and the license). See: https://creativecommons.org/licenses/by-nc-nd/4.0/.
References
- Harbeck N, Gnant M. Breast cancer. Lancet 2017;389:1134-50. [Crossref] [PubMed]
- Noguchi M, Inokuchi M, Noguchi M, et al. Axillary surgery for breast cancer: past, present, and future. Breast Cancer 2021;28:9-15. [Crossref] [PubMed]
- Bernas M, Thiadens SRJ, Stewart P, et al. Secondary lymphedema from cancer therapy. Clin Exp Metastasis 2022;39:239-47. [Crossref] [PubMed]
- McLaughlin SA, Brunelle CL, Taghian A. Breast Cancer-Related Lymphedema: Risk Factors, Screening, Management, and the Impact of Locoregional Treatment. J Clin Oncol 2020;38:2341-50. [Crossref] [PubMed]
- DiSipio T, Rye S, Newman B, et al. Incidence of unilateral arm lymphoedema after breast cancer: a systematic review and meta-analysis. Lancet Oncol 2013;14:500-15. [Crossref] [PubMed]
- Ribeiro Pereira ACP, Koifman RJ, Bergmann A. Incidence and risk factors of lymphedema after breast cancer treatment: 10 years of follow-up. Breast 2017;36:67-73. [Crossref] [PubMed]
- Rockson SG, Keeley V, Kilbreath S, et al. Cancer-associated secondary lymphoedema. Nat Rev Dis Primers 2019;5:22. [Crossref] [PubMed]
- Coriddi M, Dayan J, Sobti N, et al. Systematic Review of Patient-Reported Outcomes following Surgical Treatment of Lymphedema. Cancers (Basel) 2020;12:565. [Crossref] [PubMed]
- Warren AG, Slavin SA. Scar lymphedema: fact or fiction? Ann Plast Surg 2007;59:41-5. [Crossref] [PubMed]
- Avraham T, Clavin NW, Daluvoy SV, et al. Fibrosis is a key inhibitor of lymphatic regeneration. Plast Reconstr Surg 2009;124:438-50. [Crossref] [PubMed]
- Shi JH, Guan H, Shi S, et al. Protection against TGF-β1-induced fibrosis effects of IL-10 on dermal fibroblasts and its potential therapeutics for the reduction of skin scarring. Arch Dermatol Res 2013;305:341-52. [Crossref] [PubMed]
- Zampell JC, Yan A, Elhadad S, et al. CD4(+) cells regulate fibrosis and lymphangiogenesis in response to lymphatic fluid stasis. PLoS One 2012;7:e49940. [Crossref] [PubMed]
- Avraham T, Zampell JC, Yan A, et al. Th2 differentiation is necessary for soft tissue fibrosis and lymphatic dysfunction resulting from lymphedema. FASEB J 2013;27:1114-26. [Crossref] [PubMed]
- Zampell JC, Avraham T, Yoder N, et al. Lymphatic function is regulated by a coordinated expression of lymphangiogenic and anti-lymphangiogenic cytokines. Am J Physiol Cell Physiol 2012;302:C392-404. [Crossref] [PubMed]
- Visuri MT, Honkonen KM, Hartiala P, et al. VEGF-C and VEGF-C156S in the pro-lymphangiogenic growth factor therapy of lymphedema: a large animal study. Angiogenesis 2015;18:313-26. [Crossref] [PubMed]
- Schwager S, Detmar M. Inflammation and Lymphatic Function. Front Immunol 2019;10:308. [Crossref] [PubMed]
- Aschen S, Zampell JC, Elhadad S, et al. Regulation of adipogenesis by lymphatic fluid stasis: part II. Expression of adipose differentiation genes. Plast Reconstr Surg 2012;129:838-47. [Crossref] [PubMed]
- Uzkeser H, Karatay S, Erdemci B, et al. Efficacy of manual lymphatic drainage and intermittent pneumatic compression pump use in the treatment of lymphedema after mastectomy: a randomized controlled trial. Breast Cancer 2015;22:300-7. [Crossref] [PubMed]
- Dayan JH, Ly CL, Kataru RP, et al. Lymphedema: Pathogenesis and Novel Therapies. Annu Rev Med 2018;69:263-76. [Crossref] [PubMed]
- Barufi S, Pereira de Godoy HJ, Pereira de Godoy JM, et al. Exercising and Compression Mechanism in the Treatment of Lymphedema. Cureus 2021;13:e16121. [Crossref] [PubMed]
- Carl HM, Walia G, Bello R, et al. Systematic Review of the Surgical Treatment of Extremity Lymphedema. J Reconstr Microsurg 2017;33:412-25. [Crossref] [PubMed]
- Kayıran O, De La Cruz C, Tane K, et al. Lymphedema: From diagnosis to treatment. Turk J Surg 2017;33:51-7. [Crossref] [PubMed]
- Beederman M, Garza RM, Agarwal S, et al. Outcomes for Physiologic Microsurgical Treatment of Secondary Lymphedema Involving the Extremity. Ann Surg 2022;276:e255-63. [Crossref] [PubMed]
- Brown S, Dayan JH, Coriddi M, et al. Pharmacological Treatment of Secondary Lymphedema. Front Pharmacol 2022;13:828513. [Crossref] [PubMed]
- Nosenko MA, Drutskaya MS, Moisenovich MM, et al. Bioengineering of Artificial Lymphoid Organs. Acta Naturae 2016;8:10-23. [Crossref] [PubMed]
- Wang C, Sun W, Ye Y, et al. Bioengineering of Artificial Antigen Presenting Cells and Lymphoid Organs. Theranostics 2017;7:3504-16. [Crossref] [PubMed]
- Kanapathy M, Patel NM, Kalaskar DM, et al. Tissue-engineered lymphatic graft for the treatment of lymphedema. J Surg Res 2014;192:544-54. [Crossref] [PubMed]
- Schaupper M, Jeltsch M, Rohringer S, et al. Lymphatic Vessels in Regenerative Medicine and Tissue Engineering. Tissue Eng Part B Rev 2016;22:395-407. [Crossref] [PubMed]
- Nguyen D, Zaitseva TS, Zhou A, et al. Lymphatic regeneration after implantation of aligned nanofibrillar collagen scaffolds: Preliminary preclinical and clinical results. J Surg Oncol 2022;125:113-22. [Crossref] [PubMed]
- Weitman E, Cuzzone D, Mehrara BJ. Tissue engineering and regeneration of lymphatic structures. Future Oncol 2013;9:1365-74. [Crossref] [PubMed]
- Frueh FS, Gousopoulos E, Rezaeian F, et al. Animal models in surgical lymphedema research--a systematic review. J Surg Res 2016;200:208-20. [Crossref] [PubMed]
- Hartiala P, Saaristo AM. Growth factor therapy and autologous lymph node transfer in lymphedema. Trends Cardiovasc Med 2010;20:249-53. [Crossref] [PubMed]
- Rauniyar K, Jha SK, Jeltsch M. Biology of Vascular Endothelial Growth Factor C in the Morphogenesis of Lymphatic Vessels. Front Bioeng Biotechnol 2018;6:7. [Crossref] [PubMed]
- Rochlin DH, Inchauste S, Zelones J, et al. The role of adjunct nanofibrillar collagen scaffold implantation in the surgical management of secondary lymphedema: Review of the literature and summary of initial pilot studies. J Surg Oncol 2020;121:121-8. [Crossref] [PubMed]
- Hadamitzky C, Zaitseva TS, Bazalova-Carter M, et al. Aligned nanofibrillar collagen scaffolds - Guiding lymphangiogenesis for treatment of acquired lymphedema. Biomaterials 2016;102:259-67. [Crossref] [PubMed]
- Deptula P, Zhou A, Posternak V, et al. Multimodality Approach to Lymphedema Surgery Achieves and Maintains Normal Limb Volumes: A Treatment Algorithm to Optimize Outcomes. J Clin Med 2022;11:598. [Crossref] [PubMed]
- Nguyen DH, Zhou A, Posternak V, et al. Nanofibrillar Collagen Scaffold Enhances Edema Reduction and Formation of New Lymphatic Collectors after Lymphedema Surgery. Plast Reconstr Surg 2021;148:1382-93. [Crossref] [PubMed]
- Shuck J, Schaverien MV, Chang EI. Discussion: Nanofibrillar Collagen Scaffold Enhances Edema Reduction and Formation of New Lymphatic Collectors after Lymphedema Surgery. Plast Reconstr Surg 2021;148:1394-5. [Crossref] [PubMed]
- Huang NF, Okogbaa J, Lee JC, et al. The modulation of endothelial cell morphology, function, and survival using anisotropic nanofibrillar collagen scaffolds. Biomaterials 2013;34:4038-47. [Crossref] [PubMed]
- Baik JE, Park HJ, Kataru RP, et al. TGF-β1 mediates pathologic changes of secondary lymphedema by promoting fibrosis and inflammation. Clin Transl Med 2022;12:e758. [Crossref] [PubMed]
- Mehrara BJ, Dayan JH, Coriddi M, et al. Pathophysiology and Molecular Research in Lymphedema. In: Principles and Practice of Lymphedema Surgery. 2022.
- Kilarski WW. Physiological Perspective on Therapies of Lymphatic Vessels. Adv Wound Care (New Rochelle) 2018;7:189-208. [Crossref] [PubMed]
- Suami H, Scaglioni MF, Dixon KA, et al. Interaction between vascularized lymph node transfer and recipient lymphatics after lymph node dissection-a pilot study in a canine model. J Surg Res 2016;204:418-27. [Crossref] [PubMed]
- Cornelissen AJ, Qiu SS, Lopez Penha T, et al. Outcomes of vascularized versus non-vascularized lymph node transplant in animal models for lymphedema. Review of the literature. J Surg Oncol 2017;115:32-6. [Crossref] [PubMed]
- Honkonen KM, Visuri MT, Tervala TV, et al. Lymph node transfer and perinodal lymphatic growth factor treatment for lymphedema. Ann Surg 2013;257:961-7. [Crossref] [PubMed]
- Tammela T, Saaristo A, Holopainen T, et al. Therapeutic differentiation and maturation of lymphatic vessels after lymph node dissection and transplantation. Nat Med 2007;13:1458-66. [Crossref] [PubMed]
- Yamakawa M, Doh SJ, Santosa SM, et al. Potential lymphangiogenesis therapies: Learning from current antiangiogenesis therapies-A review. Med Res Rev 2018;38:1769-98. [Crossref] [PubMed]
- Hartiala P, Suominen S, Suominen E, et al. Phase 1 Lymfactin(®) Study: Short-term Safety of Combined Adenoviral VEGF-C and Lymph Node Transfer Treatment for Upper Extremity Lymphedema. J Plast Reconstr Aesthet Surg 2020;73:1612-21. [Crossref] [PubMed]
- Leppäpuska IM, Hartiala P, Suominen S, et al. Phase 1 Lymfactin® Study: 24-month Efficacy and Safety Results of Combined Adenoviral VEGF-C and Lymph Node Transfer Treatment for Upper Extremity Lymphedema. J Plast Reconstr Aesthet Surg 2022;75:3938-45. [Crossref] [PubMed]
- Gibot L, Galbraith T, Kloos B, et al. Cell-based approach for 3D reconstruction of lymphatic capillaries in vitro reveals distinct functions of HGF and VEGF-C in lymphangiogenesis. Biomaterials 2016;78:129-39. [Crossref] [PubMed]
- Kajiya K, Hirakawa S, Ma B, et al. Hepatocyte growth factor promotes lymphatic vessel formation and function. EMBO J 2005;24:2885-95. [Crossref] [PubMed]
- Saito Y, Nakagami H, Morishita R, et al. Transfection of human hepatocyte growth factor gene ameliorates secondary lymphedema via promotion of lymphangiogenesis. Circulation 2006;114:1177-84. [Crossref] [PubMed]
- Viitanen TP, Visuri MT, Sulo E, et al. Anti-inflammatory effects of flap and lymph node transfer. J Surg Res 2015;199:718-25. [Crossref] [PubMed]
- Sano M, Hirakawa S, Suzuki M, et al. Potential role of transforming growth factor-beta 1/Smad signaling in secondary lymphedema after cancer surgery. Cancer Sci 2020;111:2620-34. [Crossref] [PubMed]
- Ghanta S, Cuzzone DA, Torrisi JS, et al. Regulation of inflammation and fibrosis by macrophages in lymphedema. Am J Physiol Heart Circ Physiol 2015;308:H1065-77. [Crossref] [PubMed]
- Mehrara BJ, Park HJ, Kataru RP, et al. Pilot Study of Anti-Th2 Immunotherapy for the Treatment of Breast Cancer-Related Upper Extremity Lymphedema. Biology (Basel) 2021;10:934. [Crossref] [PubMed]
- Tian W, Rockson SG, Jiang X, et al. Leukotriene B(4) antagonism ameliorates experimental lymphedema. Sci Transl Med 2017;9:eaal3920. [Crossref] [PubMed]
- Rockson SG, Tian W, Jiang X, et al. Pilot studies demonstrate the potential benefits of antiinflammatory therapy in human lymphedema. JCI Insight 2018;3:e123775. [Crossref] [PubMed]
- Elborn JS, Ahuja S, Springman E, et al. EMPIRE-CF: A phase II randomized placebo-controlled trial of once-daily, oral acebilustat in adult patients with cystic fibrosis - Study design and patient demographics. Contemp Clin Trials 2018;72:86-94. [Crossref] [PubMed]
- Jia W, He W, Wang G, et al. Enhancement of Lymphangiogenesis by Human Mesenchymal Stem Cell Sheet. Adv Healthc Mater 2022;11:e2200464. [Crossref] [PubMed]
- Hu LR, Pan J. Adipose-derived stem cell therapy shows promising results for secondary lymphedema. World J Stem Cells 2020;12:612-20. [Crossref] [PubMed]
- Shukla L, Luwor R, Ritchie ME, et al. Therapeutic Reversal of Radiotherapy Injury to Pro-fibrotic Dysfunctional Fibroblasts In Vitro Using Adipose-derived Stem Cells. Plast Reconstr Surg Glob Open 2020;8:e2706. [Crossref] [PubMed]
- Zhang N, Hu L, Liu J, et al. Wnt Signaling Regulates the Lymphatic Endothelial Transdifferentiation of Adipose-Derived Stromal Cells In Vitro. Cell Reprogram 2021;23:117-26. [Crossref] [PubMed]
- Li B, Yang J, Wang R, et al. Delivery of vascular endothelial growth factor (VEGFC) via engineered exosomes improves lymphedema. Ann Transl Med 2020;8:1498. [Crossref] [PubMed]
- Ahmadzadeh N, Robering JW, Kengelbach-Weigand A, et al. Human adipose-derived stem cells support lymphangiogenesis in vitro by secretion of lymphangiogenic factors. Exp Cell Res 2020;388:111816. [Crossref] [PubMed]
- Yoshida S, Hamuy R, Hamada Y, et al. Adipose-derived stem cell transplantation for therapeutic lymphangiogenesis in a mouse secondary lymphedema model. Regen Med 2015;10:549-62. [Crossref] [PubMed]
- Takeda K, Sowa Y, Nishino K, et al. Adipose-derived stem cells promote proliferation, migration, and tube formation of lymphatic endothelial cells in vitro by secreting lymphangiogenic factors. Ann Plast Surg 2015;74:728-36. [Crossref] [PubMed]
- Ogino R, Hayashida K, Yamakawa S, et al. Adipose-Derived Stem Cells Promote Intussusceptive Lymphangiogenesis by Restricting Dermal Fibrosis in Irradiated Tissue of Mice. Int J Mol Sci 2020;21:3885. [Crossref] [PubMed]
- Strassburg S, Torio-Padron N, Finkenzeller G, et al. Adipose-Derived Stem Cells Support Lymphangiogenic Parameters In Vitro. J Cell Biochem 2016;117:2620-9. [Crossref] [PubMed]
- Maruccia M, Elia R, Ciudad P, et al. Postmastectomy upper limb lymphedema: Combined vascularized lymph node transfer and scar release with fat graft expedites surgical and patients' related outcomes. A retrospective comparative study. J Plast Reconstr Aesthet Surg 2019;72:892-901. [Crossref] [PubMed]
- Toyserkani NM, Jensen CH, Sheikh SP, et al. Cell-Assisted Lipotransfer Using Autologous Adipose-Derived Stromal Cells for Alleviation of Breast Cancer-Related Lymphedema. Stem Cells Transl Med 2016;5:857-9. [Crossref] [PubMed]
- Toyserkani NM, Jensen CH, Tabatabaeifar S, et al. Adipose-derived regenerative cells and fat grafting for treating breast cancer-related lymphedema: Lymphoscintigraphic evaluation with 1 year of follow-up. J Plast Reconstr Aesthet Surg 2019;72:71-7. [Crossref] [PubMed]
- Toyserkani NM, Christensen ML, Sheikh SP, et al. Stem cells show promising results for lymphoedema treatment--a literature review. J Plast Surg Hand Surg 2015;49:65-71. [Crossref] [PubMed]
- Toyserkani NM, Jensen CH, Andersen DC, et al. Human and Autologous Adipose-derived Stromal Cells Increase Flap Survival in Rats Independently of Host Immune Response. Ann Plast Surg 2018;80:181-7. [Crossref] [PubMed]
- Toyserkani NM, Jørgensen MG, Tabatabaeifar S, et al. Concise Review: A Safety Assessment of Adipose-Derived Cell Therapy in Clinical Trials: A Systematic Review of Reported Adverse Events. Stem Cells Transl Med 2017;6:1786-94. [Crossref] [PubMed]
- Jørgensen MG, Toyserkani NM, Jensen CH, et al. Adipose-derived regenerative cells and lipotransfer in alleviating breast cancer-related lymphedema: An open-label phase I trial with 4 years of follow-up. Stem Cells Transl Med 2021;10:844-54. [Crossref] [PubMed]
- Hu LR, Pan J. Adipose-derived stem cell therapy shows promising results for secondary lymphedema. World J Stem Cells 2020;12:612-20. [Crossref] [PubMed]
- Gao S, Ma JJ, Lu C. Prognostic significance of VEGF-C immunohistochemical expression in breast cancer: a meta-analysis. Tumour Biol 2014;35:1523-9. [Crossref] [PubMed]
- Maañón J, Perez D, Rhode A, et al. High serum vascular endothelial growth factor C predicts better relapse-free survival in early clinically node-negative breast cancer. Oncotarget 2018;9:28131-40. [Crossref] [PubMed]
- Zhang Z, Luo G, Tang H, et al. Prognostic Significance of High VEGF-C Expression for Patients with Breast Cancer: An Update Meta Analysis. PLoS One 2016;11:e0165725. [Crossref] [PubMed]
- Laukka M, Hoppela E, Salo J, et al. Preperitoneal Fat Grafting Inhibits the Formation of Intra-abdominal Adhesions in Mice. J Gastrointest Surg 2020;24:2838-48. [Crossref] [PubMed]
- Siniscalco D, Giordano C, Galderisi U, et al. Long-lasting effects of human mesenchymal stem cell systemic administration on pain-like behaviors, cellular, and biomolecular modifications in neuropathic mice. Front Integr Neurosci 2011;5:79. [Crossref] [PubMed]
Cite this article as: Rannikko E, Visuri M, Hartiala P. Breast cancer related lymphedema—novel regenerative therapies: a narrative review. Ann Breast Surg 2024;8:9.