Indocyanine green angiography—current status on quantification of perfusion: a narrative review
Introduction
Ischemia and necrosis pose significant concerns during breast reconstruction (1). Therefore, efforts have been directed towards preventing its incidence intraoperatively (1,2). Commonly employed tests encompass assessments of flap texture, colour, bleeding from flap edges, capillary refill, temperature, and ultrasonographic evaluation (3). However, these methods lack objective standardization, and their reported specificity ranges from 10–30% (4-6). While over-resecting the flap is the safest option, it inevitably leads to removal of viable skin (7). Since a high percentage of flaps can potentially be salvaged if hypoperfusion is detected early, a more sophisticated tool for assessment of tissue perfusion is critically needed (8-10).
A promising method for evaluating flap perfusion during breast reconstruction is indocyanine green fluorescence angiography (ICG-FA). Still et al. were the pioneers in evaluating the feasibility of the method for examining reconstructive flap perfusion (11). Although perfusion was evaluated qualitatively, meaning that the fluorescence intensity was subjectively interpreted, they found that the method could diagnose poor flap circulation despite a clinically satisfactory appearance (11).
ICG-FA has now become an established method for assessing tissue perfusion in various surgical specialities (12), and its potential in reducing fat and skin necrosis during breast reconstruction has been convincingly demonstrated by several studies (13-15). The introduction of quantitative software has indeed accelerated breakthroughs in this field (6), allowing several studies to establish objective cut-off values in order to predict post-operative complications (7,16-19).
However, similar studies have revealed that cut-off values differ in terms of sensitivity (ability to designate insufficiently perfused tissue as non-viable) and specificity (ability to designate sufficiently perfused tissue as viable) depending on the applied camera system, underlying software, parameter selection, and the specifically applied value that defines whether surgical revision is needed (18). Therefore, there is still no consensus on which method is most accurate for the assessment of perfusion in breast reconstruction (20).
To translate technical analysis into clinically applicable information, surgeons need insights into the methodology, i.e., how it represents the hemodynamic condition of flaps, in which context it is applicable, and the factors by which it is influenced. Therefore, this narrative review aims to provide a current overview of ICG-based quantification techniques for tissue perfusion status in breast reconstruction. We present this article in accordance with the Narrative Review reporting checklist (available at https://abs.amegroups.com/article/view/10.21037/abs-24-15/rc).
Methods
Search strategy
On the 1st of November 2023, an electronic search was conducted using the PubMed and Embase databases. When possible, medical subject headings (MeSH) were adopted. Included MeSH comprised “perfusion”, “indocyanine green”, and “optical imaging”. The employed search strategies are available for reference in Table 1.
Table 1
Items | Specification |
---|---|
Date of search | 11.01.2023 |
Databases and other sources searched | PubMed, Embase |
Search terms used | See Supplementary file (Appendix 1) |
Timeframe | Up to 1st November, 2023 |
Inclusion and exclusion criteria | Only full-text articles in English were included. Randomized controlled trials, prospective studies, cohort studies, case-control studies, and retrospective studies were included. Case reports, animal studies, and studies not providing details on the quantification method were excluded |
Selection process | The study selection process was performed independently by F.T.A. under supervision of T.E.D., utilising the well-recognized two-stage approach. First stage involved screening of titles and abstracts, followed by stage two, with full-text screening Consensus was obtained using Covidence |
Article selection
Reporting and screening were systematically performed utilising the well-recognized two-stage approach. First stage involved screening of titles and abstracts, followed by stage two, with full-text screening (Figure 1). The process was carried out using Covidence. However, the narrative review of the literature and the results obtained during this study is not part of a formally registered review.
Only full-text articles in English were included. Randomized controlled trials (RCTs), prospective studies, cohort studies, case-control studies, and retrospective studies which described the use of intra-operative or post-operative quantitative ICG-FA to evaluate tissue perfusion during mastectomy procedures were included. Reviews were screened for references. Case reports, animal studies, and studies not providing details on the quantification method were excluded. An extensive array of parameters has been investigated in animal studies, otorhinolaryngology, gastro-intestinal surgery, vascular surgery, and neurosurgery, but these studies were not included in the review.
Quality assessment
Each included article was rated according to the hierarchy of evidence, following the criteria outlined by the American Society of Plastic Surgeons (ASPS) Level of Evidence rating scale (21). A heightened level on this scale corresponds to increased reliability of findings and greater validity of the drawn results and conclusions. In case of inconsistencies between the evidence level appointed by the primary author (F.T.A.) and previously conducted reviews, the senior author (T.E.D.) was consulted for resolution.
Data collection
The following study characteristics were extracted: camera system, quantitative software, surgical set-up, ICG dosage, mastectomy type, reconstruction type, time-intensity curves, parameter, endpoint, proposed cut-off value and its associated sensitivity, specificity, negative predictive value (NPV), and positive predictive value (PPV), conclusion regarding employed parameters as tool for perfusion evaluation, and reference region of interest (ROI) selection. The data were selected independently by the primary author, but when in doubt, TED was consulted.
ICG-FA
Indocyanine green (ICG)
ICG is a well-known fluorescent dye that provides real-time information on tissue perfusion. Following intravenous injection, ICG binds chemically with plasma proteins and remains confined within the intravascular compartment during its plasma half-life of 2–4 minutes. Near-infrared camera systems, equipped with built-in excitation sources utilising laser-assisted near-infrared light to excite the dye in vivo, are subsequently employed to visualise the emitted fluorescence, which is displayed on a greyscale from 0 to 255. Thus, ICG facilitates evaluation of tissue perfusion (22-24). This approach is traditionally referred to as the qualitative methodology.
Imaging systems
Most studies within breast reconstruction have reported utilisation of various imaging devices developed by Novadac and then Stryker, for instance SPY Elite and SPY-PHI (Stryker AB, Malmö, Sweden, www.stryker.com). However, the utilisation of Quest Spectrum Platform® (Quest medical imaging, B.V., Wieringerwerf, Netherland, www.quest-mi.com), FLUOBEAM® (Fluoptics, Grenoble, France, www.fluoptics.com), Photodynamic Eye® (Hamamatsu Photonics K. K., Hamamatsu City, Japan, www.hamamatsu.com), and IC-VIEW® (Pulsion Medical Systems AG, Munich, Germany, www.getinge.com) has also been reported. Additionally, fluorescence imaging modes have been incorporated into microscopes by Carl Zeiss and Leica, facilitating the utilisation of fluorescence imaging during micro-surgeries. Portable handheld fluorescence imaging devices are widely used by reconstructive surgeons, not only intra-operatively but also in pre- and post-operative settings (25,26). An overview of commonly applied camera systems is available for reference in Table 2.
Table 2
Studies | Year | Level of evidence | Technical setup | Surgical procedure | Analysis method | |||||||||||
---|---|---|---|---|---|---|---|---|---|---|---|---|---|---|---|---|
Imaging system | Image analysis software | Dose | Working distance | Type of mastectomy | Prosthetic reconstruction | Autologous reconstruction | Reports time-intensity curves | Parameters | ||||||||
Implant | Expander | Pedicled flap | Free flap | |||||||||||||
De Lorenzi (27) | 2005 | III | IC-VIEW | IC-CALC | 0.5 mg/kg | N/R | Nipple-sparing, subcutaneous | Yes | I, T, C | |||||||
Newman (28) | 2010 | III | Spy NOS | SPY-Q | 10 mg | N/R | Skin-sparing | E | TRAM | No | R | |||||
Holm (26) | 2010 | II | Carl Zeiss and Co | N/R | 0.5 mg/kg | N/R | N/R | LD | DIEP, msTRAM, SIEA | No | T | |||||
Phillips (17) | 2012 | II | Spy 2001 | SPY-Q | 17.5 mg | N/R | Simple, modified radical | E | No | I, R | ||||||
Moyer (7) | 2012 | III | Spy Elite | SPY-Q | 5 cc bolus | 20 cm | Skin-sparing | E | LD | TRAM | No | I, R | ||||
Newman (16) | 2013 | III | Spy Elite | SPY-Q | 10 mg | N/R | N/R | E | No | I, R | ||||||
Sood (29) | 2013 | IV | Spy NOS | SPY-Q | 3 cc | N/R | N/R | I | E | No | R | |||||
Wapnir (30) | 2014 | IV | Spy Elite | SPY-Q | 7.5 mg | N/R | Nipple-sparing | E | DIEP | Yes | N/A | |||||
Munabi (18) | 2014 | II | Spy Elite | SPY-Q | 10 mg | N/R | Simple or radical skin-sparing, nipple-sparing | I | E | TRAM | DIEP | No | I | |||
Phillips (31) | 2014 | IV | Spy Elite and Spy 2001 | SPY-Q | 10 mg | N/R | Prophylactic, therapeutic | E | TRAM | I | ||||||
Ludolph (32) | 2016 | IV | Spy Elite | SPY-Q | 10 mg | N/R | N/A | E | DIEP, msTRAM, | No | R | |||||
Mattison (33) | 2016 | IV | Spy Elite | SPY-Q | N/R | N/R | Skin-sparing | I | E | No | I | |||||
Hitier (34) | 2016 | II | Fluobeam | Fluobeam v1.47 | 0.025 mg/kg | 20 cm | N/R | DIEP | Yes | I, T, C | ||||||
Gorai (19) | 2017 | II | Photo-dynamic Eye | Hamamatsu Photonics | 25 mg | 30 cm | Total | E | Yes | R, T, C | ||||||
Alstrup (35) | 2018 | IV | Spy Elite | SPY-Q | 7.5 mg | N/R | N/R | LD, msLD | TRAM | No | R | |||||
Hammer-Hansen (36) | 2018 | III | Spy Elite | SPY-Q | N/R | N/R | Skin-sparing | I | E | No | R | |||||
Mirhaidari (37) | 2018 | II | Spy Elite | SPY-Q | 7.5 mg | N/R | Nipple-sparing or nipple-sacrificing | I | E | LD, TRAM | NOS | No | I | |||
de Vita (38) | 2018 | IV | Quest Spectrum Platform | Spectrum Capture Suite | 0.2 mg/kg | 20 cm | Nipple-sparring | I | No | N/R | ||||||
Yang (39) | 2018 | III | Spy Elite | Spy-Q | 3 cc | N/R | Modified radical, skin-sparing, nipple-sparing | E | Yes | C | ||||||
Wang (40) | 2018 | III | SPY NOS | Spy-Q | 7.5 mg | N/R | Nipple-sparring | Yes | C | |||||||
Girard (41) | 2019 | II | Spy Elite | SPY-Q | 5 mg | N/R | – | DIEP | No | I, C | ||||||
Kim (42) | 2019 | III | Spy NOS | Spy-Q | 15 mg | N/R | Nipple-sparing | I | E | Yes | R | |||||
Varela (43) | 2020 | I | Photo-dynamic Eye | IC-CALC 2.0 | 0.2 mg/kg | N/R | – | DIEP | Yes | R | ||||||
Ogawa (44) | 2021 | III | Spy SP3000 | SPY-Q | 0.05 mg/kg | 30 cm | Without reconstruction |
Yes | I, R, C | |||||||
Van Den Hoven (20) | 2022 | II | Quest spectrum platform | Quest Research Framework | 7.5 mg | 50 cm | – | DIEP, PAP, SIEA | Yes | T, C | ||||||
Mastronardi (45) | 2022 | II | SPY-PHI | ImageJ | 0.2 mg/kg | N/R | Nipple-sparing, skin-reducing or skin-sparing | I | E | Yes | T, I, R | |||||
Pruimboom (46) | 2023 | III | Fluobeam 800 | ImageJ | 0.1 mg/kg | 25 cm | Skin-sparing | DIEP, DUG | T, I | |||||||
Lauritzen (47) | 2023 | II | Spy Elite and SPY-PHI | SPY-Q and SPY-QP | 2.5 mg/mL | 20 and 10–40 cm | – | I | LD | DIEP | No | R | ||||
Choudhary (48) | 2023 | I | Spy Elite | SPY-Q | 7.5–10 mg | N/R | N/R | DIEP | No | R |
NOS, not otherwise specified; N/R, not reported; N/A, not applicable; TRAM, transverse rectus abdominis myocutaneous flap; LD, latissimus dorsi muscle flap; msLD, muscle-sparing latissimus dorsi muscle flap; DIEP, deep inferior epigastric artery perforator; msTRAM, muscle-sparing free transverse rectus abdominis myocutaneous flap; SIEA, superficial inferior epigastric artery flap; PAP, profunda artery perforator flap; DUG, diagonal upper gracilis; I, intensity-dependent parameter; T, time-related parameter; C, combined parameter; R, relative parameter.
Quantitative imaging
Despite the diversity in employed imaging systems, there is a growing consensus to integrate quantitative software into the methodology, motivated by the subjective nature of the qualitative methodology (49). The software plays a pivotal role in translating fluorescence intensity into objective measures, providing numerical values instead of displaying shades of grey. Thereby, numerical outputs facilitate the establishment of objective cut-off values that can be used as thresholds. Some software employs machine learning algorithms for real-time signal processing, incorporating a motion correction algorithm to track the selected tissue and mitigate the impact of movement (50), as this has challenged the utility of quantitative imaging in free flap procedures (51). Our review identified 29 papers reporting on quantitative software application within breast reconstruction (Table 2).
Software selection
Several imaging systems offer build-in quantitative software, including SPY-Q® (16,29,44) and SPY-QP® (Stryker AB, Malmö, Sweden) (47), Quest Research Framework® (Quest medical imaging, B.V., Wieringerwerf, The Netherland) (20,52), EleVisionTM (Medtronic, Dublin, Ireland, www.medtronic.com) (53), IC-CALC® (Pulsion Medical Systems AG, Munich, Germany) (54), U11437 software® (Hamamatsu Photonics K. K., Hamamatsu City, Japan) (19,55), Flow800 module® (Carl Zeiss Meditec AG, Oberkochen, Germany, www.zeiss.com) (56,57), and FLUOBEAM® (Fluoptics, Grenoble, France) (34) (Table 2). Moreover, innovative and commercially available custom software solutions, such as PerfusionWorks® (Perfusion Tech Aps, Copenhagen, Denmark, www.perfusiontech.com) (50) and ImageJ (US National Institutes of Health, Bethesda, MD, USA, http://www.imagej.net) offer a more extensive array of parameters (please see below) and are compatible with most imaging systems.
Methodology
The quantitative software facilitates the selection of multiple size-adjustable ROIs and provides perfusion status information for each region. Fluorescence intensity is recorded over a defined time interval, allowing the average pixel intensity in arbitrary units (AU) to be plotted against time, generating time-intensity curves (20,44) (Figure 2). Perfusion parameters are then extrapolated, processed, and presented.
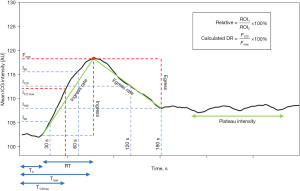
Quantitative outputs
Four distinct groups of parameters have been frequently investigated and used as definitive quantitative outputs in breast reconstruction (Tables 2-6).
Table 3
Studies | Endpoint | Technical setup | Analysis method | Accuracy | Conclusion regarding parameter selection | |||||||||
---|---|---|---|---|---|---|---|---|---|---|---|---|---|---|
Imaging system | Image analysis software | Dose | Working distance | Parameter | Cut-off (AU) | Sensitivity (%) | Specificity (%) | PPV (%) | NPV (%) | |||||
Phillips (17) | PN or FTN | Spy 2001 | SPY-Q | 17.5 mg | N/R | I120 | 3.7 | 90 | 100 | 56 | 88 | Absolute values should be favoured over relative values. Suggested using 3.7 as cut-off | ||
8.0 | 100 | 70 | – | – | ||||||||||
Moyer (7) | Necrosis | Spy Elite | SPY-Q | 5 cc | 20 cm | I60 | 6.3 | Not calculated due to insignificant difference | Absolute values appeared less trustful than relative values | |||||
12.3 | ||||||||||||||
Newman (16) | Necrosis | Spy Elite | SPY-Q | 10 mg | N/R | I30 | 18.5 | Not calculated due to insignificant difference | Relative values were more objective and reproducible compared to absolute values | |||||
25 | ||||||||||||||
Munabi (18) | Necrosis | Spy Elite | SPY-Q | 10 mg | N/R | I90 | ≤7 | 88 | 83 | 44 | 98 | Absolute values should be preferred, using an absolute cut-off value ≤7 | ||
≤13 | 100 | 72 | 35 | 100 | ||||||||||
≤7† | 83 | 97 | 83 | 98 | ||||||||||
Phillips (31) | Necrosis | Spy Elite | SPY-Q | 10 mg | N/R | I120 | 23.8 | 90 | 100 | – | – | Absolute values ≤23.8 predicted necrosis while ≥36.6 predicted viability | ||
36.6 | 100 | 70 | – | – | ||||||||||
Mattison (33) | Necrosis | Spy Elite | SPY-Q | 7.5 mg | N/R | I120 | 10 | 100 | 68.1 | 34.8 | 100 | Suggested using an absolute cut-off value of ≤10 | ||
15 | 100 | 51.1 | 25.8 | 100 | ||||||||||
20 | 100 | 27.7 | 19 | 100 | ||||||||||
Hitier (34) | Skin paddle necrosis, venous thrombosis | Fluobeam | Fluobeam v1.47 | 0.025 mg/kg | 20 cm | Ingress | 33.45 | N/R | Suggested a per-operative cut-off value of ingress of 33.45 | |||||
Mirhaidari (37) | SFN | Spy Elite | SPY-Q | 7.5 mg | N/R | I180 | >30 | N/A | ICG-FA decreased the rate of implant loss, skin flap necrosis, infection, and overall reoperation | |||||
16–30 | ||||||||||||||
10–15 | ||||||||||||||
<10 | ||||||||||||||
Girard (41) | Perfusion pattern alterations | Spy Elite | SPY-Q | 5 mg | N/R | Ingress | N/A | N/A | Ingress may refine the results when assessing DIEP flap perfusion | |||||
Ogawa (44) | Skin necrosis | SPY SP3000 | SPY-Q | 0.05 mg/kg | 30 cm | I100 | Not calculated due to insignificant difference | Fmax was less accurate than absolute intensity at 100 s, but relative values should be preferred | ||||||
Fmax | ||||||||||||||
Mastronardi (45) | PN or FTN | SPY-PHI | ImageJ | 0.2 mg/kg | N/R | FMax | 68.2 | 57 | 77 | 36 | 89 | Absolute values appear inferior compared with relative values | ||
FMin | Not calculated due to insignificant difference | |||||||||||||
Pruimboom (46) | Perfusion before and after IMA clamping | Fluobeam 800 | ImageJ | 0.1 mg/kg | 25 cm | I60 | N/A | N/A | ||||||
I90 | ||||||||||||||
I120 |
†, after excluding patients with a smoking history and patients who had an epinephrine-containing tumescent solution used during mastectomy. PN, partial necrosis; FTN, full-thickness necrosis; SFN, skin flap necrosis; IMA, internal mammary artery; N/R, not reported; AU, arbitrary units; N/A, not applicable; PPV, positive predictive value; NPV, negative predictive value; ICG-FA, indocyanine green fluorescence angiography; DIEP, deep inferior epigastric artery perforator.
Table 4
Studies | Endpoint | Analysis method | Accuracy | Conclusion regarding parameter selection | |||||
---|---|---|---|---|---|---|---|---|---|
Parameter | Cut-off | Sensitivity (%) | Specificity (%) | PPV (%) | NPV (%) | ||||
Holm (26) | Postoperative flap related complications | ITT | ≥50 s | 92 | 78 | ITT predicted development of flap compromise and early re-exploration surgery | |||
Hitier (34) | Skin paddle necrosis, venous thrombosis | ITT | N/R | ITT and T0 did not predict flap outcome | |||||
T0 | |||||||||
Gorai (19) | Mastectomy flap necrosis | TMax | Not evaluated due to study design | TMax and T1/2Max were significantly shorter in viable tissue. RT showed no significant difference | |||||
T1/2Max | |||||||||
RT | |||||||||
Van Den Hoven (20) | Time-intensity curve pattern | TMax* | Not evaluated due to study design | TMax* and TMax_Slope may reflect perfusion | |||||
TMax_Slope | |||||||||
Mastronardi (45) | Necrosis | T0 | Not reported due to insignificant difference | T1 was significantly longer in necrosis group. No statistical significance was observed for T0 and TMax | |||||
TMax | |||||||||
T1 | ≥170 s | 100 | 68 | 41 | 100 | ||||
Pruimboom (46) | Perfusion alterations before and after IMA clamping | T0 | N/A | N/A |
ITT, intrinsic transit time; T0, time interval from injection until initial flap perfusion; TMax, time from ICG injection to maximum absolute fluorescence intensity; T1/2Max, time from ICG injection to 50% maximum absolute fluorescence intensity; RT, time delay from onset of fluorescence intensity increase until maximum intensity; TMax*, time from onset of fluorescence intensity increase until maximum intensity; TMax_Slope, time from initial fluorescence increase until maximum slope value; T1, time interval from injection until perfusion in least vascularized area; IMA, internal mammary artery; N/R, not reported; N/A, not applicable; PPV, positive predictive value; NPV, negative predictive value.
Table 5
Studies | Endpoint | Analysis method | Accuracy | Conclusion regarding parameter selection | |||||
---|---|---|---|---|---|---|---|---|---|
Parameter | Cut-off | Sensitivity (%) | Specificity (%) | PPV (%) | NPV (%) | ||||
Hitier (34) | Skin paddle necrosis, venous thrombosis | Slope | N/R | Suggested slope and ingress to be more reliable than time-related parameters (T0, ITT) | |||||
Gorai (19) | Mastectomy flap necrosis | Slope_TMax | 0.2 | 91.3 | 73.8 | – | – | Slope_T1/2Max and slope_TMax provided the best correlation. No significant correlation was observed for slope_RT | |
Slope_T1/2Max | 0.4 | 92.5 | 76.9 | – | – | ||||
Slope_RT | Not calculated | ||||||||
Wang (40) | Perfusion pattern alterations | Ingress rate | N/A | Egress rate ≤2 signify that the implant needs to be downsized to avoid decreased NAC perfusion | |||||
Egress rate | |||||||||
Yang (39) | Perfusion pattern alterations | Ingress rate | N/A | Ingress rate should be the primary parameter used for implant-sizing | |||||
Egress rate | |||||||||
Girard (41) | Perfusion pattern alterations | Ingress rate | N/A | Ingress rate may refine the results when assessing DIEP flap perfusion | |||||
Ogawa (44) | Skin necrosis | Ingress rate Slope_I100 |
Relative values are preferable |
TMax, time from ICG injection to maximum absolute fluorescence intensity; T1/2Max, time from ICG injection to 50% maximum absolute fluorescence intensity; RT, time delay from onset of fluorescence intensity increase until maximum intensity; N/R, not reported; N/A, not applicable; PPV, positive predictive value; NPV, negative predictive value; T0, time interval from injection until initial flap perfusion; ITT, intrinsic transit time; NAC, nipple-areolar complex; DIEP, deep inferior epigastric artery perforator.
Table 6
Studies | Endpoint | Analysis method | Accuracy | Conclusion regarding parameter selection | ||||||
---|---|---|---|---|---|---|---|---|---|---|
Placement of reference-ROI | Parameter | Cut-off (%) | Sensitivity (%) | Specificity (%) | PPV (%) | NPV (%) | ||||
Newman (28) | Wound-healing complications | N/R | I30 | 35–45 | 100 | 91 | N/A | N/A | Relative values predict outcome with 100% sensitivity, 91% specificity, and a false positive rate of 9% | |
Phillips (17) | Necrosis | N/R | I120 | 15.6 | 90 | 50 | 56 | 88 | Absolute perfusion score was more reliable. However, 15.6% predicted necrosis. 33.7% predicted viability | |
Moyer (7) | Necrosis | Not clear | I60 | 33 | 84.6 | 87.5 | 88 | 16 | A cut-off perfusion score of 33% was useful. However, between 25% and 45% the viability remains in question | |
Newman (16) | Necrosis | Medial margin of the inframammary fold | I30 | 25.2 | – | – | – | – | Preferred relative values due to comparative values across patients. However, between 25% and 45% the viability remained in question | |
43.3 | – | – | – | – | ||||||
Gorai (19) | Mastectomy flap necrosis | Control kit | I120 | 34 | 83.8 | 98.5 | – | – | Combined parameters were more precise. However, a relative value of 34% was also feasible | |
Alstrup (35) | Major and minor complications | Lower-sternal border | I60 | 33 | N/A | Utilisation of 33% Fmax cut-off value significantly decreased major complication rate | ||||
Hammer-Hansen (36) | Necrosis | Lower-sternal border | I60 | 33 | N/A | Utilisation of 33% Fmax cut-off value did not change necrosis rate | ||||
Kim (42) | NAC necrosis requiring surgical debridement and revision within 1 month and partial NAC necrosis | Highest rate in the image was converted to 100% reference | Plateau phase | ≤10 | 80 | 100 | – | – | ≤10% predicted NAC-necrosis requiring surgical debridement and revision within 1 month in IIBR. ≤13% predicted NAC-necrosis requiring surgical debridement and revision within 1 month in DTIR. >10–≤13% predicted high risk for partial NAC-necrosis in both reconstruction types | |
≤13 | 75 | 100 | – | – | ||||||
≤13 | 85.71 | 95.12 | – | – | ||||||
Varela (43) | Fat necrosis | Perforator entry point | Slope ratio | N/A | – | – | – | – | ICG-FA reduced rate of fat necrosis, partial necrosis, and reoperation rate | |
Ogawa (44) | Skin necrosis | Caudal side of the wound with no clinically apparent surgical invasion | I100 | 12.5 | – | – | – | – | Preferred relative values. 11.2% predicted necrosis while 27.6% predicted viability. Fmax and absolute intensity at 100s was less accurate | |
27.6 | – | – | – | – | ||||||
Fmax | N/R | |||||||||
Mastronardi (45) | Partial and full-thickness necrosis | Best vascularized area within the mastectomy flap (ICG-Q0) | ICG-Q% | ≤35.6 | 57 | 81 | 40 | 89 | ICG-Q% (relative value) is a better predictor than ICG-Q1 (absolute value) and may predict necrosis | |
Lauritzen (47) | Skin flap necrosis, flap necrosis, fat necrosis, loss of reconstruction, seroma, small hematoma | Sternum/thorax/adjacent healthy tissue | N/R | 33% SPY-QP | 50 | 77 | 25 | 91 | SPY-Q software is more accurate than SPY-QP software when using the 33% cut-off | |
33% SPY-Q | 50 | 100 | 100 | 93 |
NAC, nipple-areolar complex; ROI, region of interest; N/R, not reported; N/A, not applicable; ICG-Q%, the relative intensity ratio at Tmax between ICG-Q0 and ICG-Q1; PPV, positive predictive value; NPV, negative predictive value; IIBR, immediate implant-based reconstruction; DTIR, direct-to-implant reconstruction; ICG-FA, indocyanine green fluorescence angiography.
Intensity-dependent parameters are derived from the y-axis and quantify absolute fluorescence intensity in AU. Time-related parameters are derived from the x-axis by measuring the time in seconds to specific capillary events. Combined parameters quantify the fluorescence intensity per time (AU/s) and are collected by dividing intensity-dependent parameters by their corresponding time-related parameters. Relative parameters are derived by division of two parameters of similar nature, creating an index of perfusion (Figure 2).
Time-intensity curve
Dividing the time-intensity curve into four distinct phases based on the ICG flow pattern within the ROI provides an advantageous approach for understanding the array of perfusion parameters (Figure 3). Therefore, the following sections will elucidate the time-intensity curve phases and outline the technical background of the most important individual parameters. An overview of abbreviations is provided in Supplementary file (Appendix 2).
Phase 0
Phase 0 encompasses preparation. The camera is positioned at a fixed distance, typically ranging from 25–50 cm (Table 2), and oriented with a perpendicular angulation to the tissue of interest. ICG is diluted into sterile water. Dosages ranging from 5–25 mg per injection have primarily been used, but newly available imaging systems enable the utilisation of micro-doses of 0.05–0.2 mg/kg (Table 2). If necessary, the operation room is cleared for ambient light, and ROIs are placed at the areas of interest.
Phase 1
Phase 1 corresponds to the intravenous injection of ICG. Fluorescence intensity remains static at baseline since the injected ICG bolus has not yet reached the flap. The time from injection to the onset of fluorescence intensity rise (T0) can be measured in seconds (34,45,46,58). T1 specifically defines the time from injection to initial perfusion of the least vascularized area. Route of administration may influence T0 and T1. The bolus is most commonly injected via peripheral venous catheters followed by a saline flush (15,16,18,43,44), but administration via central venous catheters has also been described (26).
Phase 2
Phase 2 (the arterial phase) represents the ICG inflow. As the ICG bolus gradually permeates the flap, the fluorescence intensity steeply rises until it reaches its peak, termed Fmax (44,45). Ingress is determined by subtracting the baseline intensity from the maximum intensity (Fmax − Fbaseline) (41,44). Thus, Fmax and ingress quantify intensity according to a distinct perfusion event, maximum intensity (dynamic analysis) (17,46). However, intensity-dependent parameters have mainly been assessed according to pre-established time points within the inflow phase (static analysis), such as intensity after 30s or 60s (I30, I60).
Tmax measures the time delay from either injection (19,27,58) or onset of intensity rise (59) to Fmax. Since rise time (RT) defines the duration of the intensity rise, we recommend using the first definition. Thereby, RT is primarily influenced by local hemodynamics since it is calculated by subtracting T0 from Tmax (56), in contrast to Tmax, which is affected by the time taken for the ICG bolus to reach peripheral tissue after leaving the heart. T1/2max measures the time until 50% of maximum intensity is reached (55).
Combined parameters include slope, defining the average ROI intensity per second (Fmax/Tmax) (19,27,43), and ingress rate [(Fmax-Fbaseline)/Tmax] (39-41,44).
Phase 3
Phase 3, or the plateau, represents the outflow of the ICG bolus and is termed the venous phase. When the bolus gradually passes the ROI, the curve exhibits a corresponding decline, signifying the venous drainage from the ROI. Thus, prolonged, and diminished wash-out may indicate venous congestion.
Intensity-dependent parameters have typically been measured according to specific time points representing the venous phase, such as I120, which represent the intensity after 120 s (Table 3). Egress represents the difference between maximum intensity and final intensity (Fmax – Ffinal) (39,40,44).
However, the intensity decline can be also measured within a truncated time interval and divided by the duration of the interval to minimize the measurement duration, which is termed egress rate.
The drainage ratio (DR) has been sporadically investigated and awaits further validation (60). DR is calculated by dividing the absolute intensity 120 s post-injection by Fmax and multiplying by 100 [(F120/Fmax) ×100].
Application of intensity-dependent parameters
Twelve studies explored intensity-dependent parameters in breast reconstruction (Table 3), of which nine correlated absolute values with post-operative flap necrosis (7,16-18,31,33,37,45,58). Three studies investigated autologous flaps (34,41,46), five studies investigated mastectomy skin flaps (16,30,42,50,51), and four studies examined both flap types together (7,18,31,37).
Technical setup
The SPY Elite and SPY-Q software were the more commonly used appliances. The working distance ranged from 20 to 30 cm, and the dose ranged from 5 to 17.5 mg per injection. Intensity was mainly measured at a specific time point after injection (static analysis), possibly due to the widespread use of the SPY Elite and SPY-Q software, offering an easily operated single time-frame image intensity analysis. Only four studies employed dynamic analysis, with two suggesting cut-off values (34,45).
Static fluorescence analysis
Munabi et al. (18) evaluated the outcomes of 48 tissue expander reconstructions and twelve autologous flap breast reconstructions [six pedicled transverse rectus abdominis myocutaneous (TRAM) flaps and six free deep inferior epigastric artery perforator (DIEP) flaps] (18). The SPY Elite and SPY-Q-software were used, with analyses based on images taken at 90s post-ICG injection. The results indicated that a fluorescence intensity value of ≤7 predicted flap necrosis with a sensitivity and specificity of 88% and 83%, respectively. Similar studies using the SPY Elite have proposed cut-off values of ≤6.3 (7), ≤10 (33), ≤18.5 (16), and ≤23.8 (31) for predicting necrosis, while values ≥12.3 (42), ≥25.0 (16), and ≥36.6 (31) were predictive of flap viability (Table 3). Lower cut-off values were suggested in a study evaluating the outdated SPY 2001 imaging system (17).
Four studies using static analysis reported the sensitivity and specificity, with three providing details on NPVs and PPVs (Table 3). Phillips et al. (31) suggested a cut-off value of 23.8 to predict necrosis with a specificity and sensitivity of 70% and 100%, respectively. If a higher cut-off value was designated, specificity decreased while sensitivity increased. Likewise, the NPVs were higher than the PPVs, indicating a tendency of this parameter group to overestimate the amount of non-viable tissue.
Dynamic fluorescence analysis
Mastronardi et al. (45) employed dynamic analysis in 34 consecutive women undergoing implant-based reconstruction using the SPY-PHI camera and ImageJ instead of the inherent SPY-Q software (45). No specific cut-off was proposed, but the assessment of Fmax offered a sensitivity of 57% and a specificity of 77% (45). The same author recently suggested an Fmax cut-off value of <66.5 to predict mastectomy skin flap necrosis with a sensitivity of 63% and a specificity of 79% (58). However, relative parameters showed greater correlations with flap outcomes. Hitier et al. (34) suggested an ingress cut-off value of 33.45 to predict flap outcome when FLUOBEAM was used to assess DIEP flap perfusion.
Evaluation
Analysis of the venous phase appears superior compared with the arterial phase, but significant variations in designated cut-off values exist across the studies. These differences likely stem from the sensitivity of absolute intensity to ambient light, camera system, angulation, working distance, dosage, time framing, albumin-concentration, and hemodynamics (12,16,44). Thorough consultation of the literature is necessary to determine how these variables should be standardized for optimal result interpretation. Although intensity-dependent parameters are suboptimal, this approach could offer a reliable quantification of perfusion on an individual basis. However, at present, relative parameters (see below) appear to provide a superior option.
Application of time-related parameters
Four studies evaluated the potential of time-related parameters to predict flap outcome or describe perfusion changes in reconstructive flaps (Table 4).
Mastectomy skin flaps
Gorai et al. (19) found Tmax and T1/2max to be significantly lower in viable tissue compared with non-viable tissue, while no statistical difference was observed for RT. However, the most interesting finding was a study investigating T1, T0, and Tmax for evaluating 38 mastectomy skin flaps in patients undergoing immediate breast reconstruction (45). Patients with superficial and full-thickness necrosis were categorized into group 2, while patients without were categorized into group 1. A high T1 value was associated with necrosis (P=0.001), and ROC-analysis disclosed the most optimal T1 cut-off value to be ≥170 s, providing 100% sensitivity, 68% specificity. No significant differences were observed for T0 and Tmax (45).
Autologous reconstruction
Van Den Hoven et al. (20) analyzed two time-related parameters in DIEP flaps, superficial inferior epigastric artery (SIEA) flaps, and profunda artery perforator (PAP) flaps. ROIs were placed at the perforator, at normally perfused tissue, at tissue with questionable perfusion, and with low perfusion. Data analysis revealed comparable values of Tmax_Slope between the perforator and normally perfused tissue, while significantly higher values were observed in flap areas of questionable perfusion and low perfusion. Tmax and Tmax_Slope were both prolonged in low-perfused regions (20).
Holm et al. (26) employed the intrinsic transit time (ITT) defined as time needed for ICG to circulate from arterial (t1) to venous anastomosis (t2) as a prognostic indicator for the success of free flaps. Their findings revealed that an ITT ≥50 s was predictive of flap necrosis, providing sensitivity and specificity rates of 92% and 98%, respectively. In a related investigation, Hitier et al. (34) investigated ITT, slope, and ingress. They disclosed that well-perfused flaps exhibited a shorter ITT, a more pronounced slope, and a higher ingress compared with flaps associated with post-operative complications.
Evaluation
While intensity-dependent parameters are well-investigated, the potential of time-related parameters remains unexplored. Therefore, studies investigating their correlation with flap outcome are needed to validate their potential and establish clinically applicable cut-off values.
Application of combined parameters
Four studies explored the use of combined parameters in breast reconstruction (Table 5).
Mastectomy skin flaps
Gorai et al. (19) assessed the efficacy of slope at Tmax, at T1/2max, and at RT in predicting flap necrosis. The strongest correlation was found for slope at T1/2max with a cut-off value of 0.4, yielding a sensitivity and specificity of 92.5% and 76.9%, respectively. A similar correlation was found for slope at Tmax, while no significance was observed for slope at RT. The study concluded that combined parameters exhibit higher sensitivity compared with relative parameters based on intensity captured at 120 s post-injection.
Wang et al. (40) showed that infraareolar incision should be preferred over supra-areolar incision due to higher ingress rate and egress rate. Furthermore, the study found the parameters applicable for sizing the implant. Yang et al. (39) examined ingress rates and egress rates across three distinct volumes in tissue expanders. The original assessment, determined by the maximum tension observed without skin bleaching after placing a tissue expander, served as the baseline (V100). Subsequently, an analysis was conducted for 50% (V50) and 150% (V150) inflation levels. The ingress values exhibited a decline from 95.4 to 73.6 to 52.1 for V50, V100, and V150, respectively, accompanied by corresponding ingress rates of 6.4, 4.9, and 3.5. Similarly, egress values decreased from 52.4 to 42.8 to 36.4, with egress rates of 0.4, 0.3, and 0.2. Based on this finding, the author stated that ingress rate should be the primary parameter used, favouring analysis of the arterial phase.
Autologous reconstruction
One study assessed various perfusion patterns in DIEP flaps and found that the mean ingress rate was significantly higher in perforator regions compared with more distal flap regions. Furthermore, the mean ingress rate was significantly impaired in patients with diabetes mellitus (41). Hitier et al. (34) revealed significantly lower post-operative slopes (P=0.02) in DIEP flaps with vascular complications compared with well-perfused flaps. An important finding was that slope and ingress came back to normal after salvage surgery. Mean values per day of slope and amplitude increased during the recovery phase in uncomplicated flaps and in revised flaps. The study concluded that slope and ingress were more reliable than time-related parameters (T0, ITT).
Application of relative parameters
Advantage of relative parameters
At least two ROIs are selected when using relative parameters. The reference ROI is placed within an unmanipulated area with normal perfusion, assigned as 100% perfused. The target ROIs are placed in the areas of interest. The outputs of the target ROIs are then provided as percentages to the reference. Thus, relative parameters are designed to be independent of conditional and inter-individual variability, which establish their theoretical advantage, but only if the reference ROI is exposed to the same factors as the target ROIs and representable for normal perfusion (16). Furthermore, the relative index is easy to interpret, also indicated by the numerous studies that have documented its usage.
Twelve studies explored relative parameters in breast reconstruction (Table 6). Seven studies investigated their correlation with mastectomy skin flap outcome (16,17,19,36,42,44,45), two studies investigated autologous flaps (35,43), and three studies examined both flap types together (7,28,47).
Mastectomy skin flaps
Moyer et al. (7) established a robust framework when they proposed using a 33% cut-off value based on intensity captured at 60 s post-injection to predict flap viability based on Gaussian distribution analysis. Areas with ≤25% perfusion were non-viable in 90% of the cases, while areas with ≥45% survived in 98%. Theoretically, this cut-off value provided a sensitivity and specificity of 84.6% and 87.5%, respectively. However, a grey zone was found between 25% and 45% perfusion, in which the viability potential remained unpredictable.
These findings were supported by Newman et al. (16), who used intensity captured at 30s post-injection and the medial margin of the inframammary fold as the reference area. The mean relative value in necrotic tissue was 25.2%, while viable tissue exhibited a mean value of 43.3%. Values of 37–45% were observed in the wound edges of skin necrosis, fitting well with the findings by Moyer et al. (7) that tissue areas with perfusion scores above 45% had a high likelihood of surviving. Gorai et al. (19) employed a control kit as reference and relative intensity captured at 120 s post-injection and suggested using a cut-off value of 34% to predict mastectomy flap necrosis, offering a sensitivity and specificity of 83.8% and 98.5%, respectively, confirming the results by Moyer et al. (7).
Mastronardi et al. (45) used the best-vascularized area within the mastectomy skin flap as reference. Although the reference ROI was placed in the operated area, the study suggested a cut-off value of 35.6% to provide a sensitivity and specificity of 57% and 81%, respectively. Thus, the 33% cut-off value has been successfully reproduced using relative intensity captured during both the arterial and venous phase and with different placement of the reference-ROI.
A few studies have not been able to reproduce or benefit from applying the 33% cut-off value.
Hammer-Hansen et al. (36) did not disclose statistical significance between groups when the 33% cut-off value was used to guide the excision of hypoperfused tissue in patients undergoing implant-based immediate breast reconstruction. However, the study may have been insufficiently powered to disclose such associations. Ogawa et al. (44) found that the relative value of intensity captured at 100 s (plateau phase) was more accurate than Fmax (arterial phase) in predicting mastectomy skin flap necrosis, favouring analyses of the plateau phase. Furthermore, the study found the lowest mean relative rates for the necrosis group to be 11.2% while a value of 27.6% predicted viability, proposing to use a 12.5% cut-off. The slightly lower values may be explained by the absence of prosthetic reconstruction. Comparable values were found by Kim et al. (42), possibly explained by the placement of the reference-ROI in the highest rate of the image.
Autologous reconstruction
In a retrospective study, Alstrup et al. (35) utilised the designated 33% cut-off value to guide intra-operative decision-making on the excision of hypoperfused tissue during unilateral or bilateral pedicled autologous flap breast reconstruction. However, no significant differences regarding skin necrosis rate were found between patients with or without utilisation of ICG-FA.
Varela et al. (43) conducted an RCT wherein qualitative ICG-FA was used to guide flap trimming during DIEP-based free flap breast reconstruction. The use of qualitative ICG-FA led to a reduction in the incidence of fat necrosis and partial flap loss reoperations from 59.3% to 8.3% and 18.2% to 0%, respectively. A retrospective evaluation of the relative ratio of slopes with the reference ROI placed at the perforator showed a significant difference between the groups. However, the study did not correlate specific slope ratios with flap outcome. Thus, in this context, the study can only be used to pinpoint a promising parameter, which currently await further investigation.
Lauritzen et al. (47) recently conducted a comparative and observational small scale study, examining the efficacy of SPY-Q (SPY Elite) versus SPY-QP (SPY-PHI) in predicting per- and post-operative complications among sixteen patients undergoing a total of 20 breast reconstructions. SPY-Q demonstrated superior predictive performance compared with SPY-QP when employing the 33% cut-off value. However, the manufacturer has declared that SPY-QP operates independently of tissue contour, possibly indicating that the software is based on a distinct set of parameters from SPY-Q. This could be combined parameters, necessitating the establishment of new cut-off values tailored to relative parameters derived from combined parameters.
Evaluation
The utilisation of relative parameters based on intensity, employing a 33–34% cut-off, represents the most well-documented option. However, the relative index of intensity may be susceptible to biases caused by camera angulation and distance, and user-dependent variations may arise as the machine operator selects an area to serve as a 100% perfusion reference point to which all other parameters are compared standardized (18). Moreover, diffusion/retrograde flow can distort the intensity in the target ROI, falsely increasing the signal. In theory, this will only influence the intensity-dependent parameters. Therefore, relative parameters based on combined and time-related parameters may be less prone to biases and warrants further investigation.
Application of time-intensity curve patterns
Wapnir et al. (30) described three different time-intensity curve patterns of post-mastectomy nipple-areolar complex (NAC) perfusion based on whether the perfusion appeared to predominantly arise from the underlying breast tissue (V1), the surrounding tissue (V2), or a combination (V3). This was accomplished by tracing the perfusion of the nipple, the areola, and the surrounding skin. The study disclosed that the V1 pattern was significantly associated with ischemia (30). Similar findings were presented in another study that used Tmax, slope, and Fmax to describe the perfusion changes of the NAC after nipple-sparing mastectomy (27).
ICG-FA and breast reconstructive procedures
Although quantitative ICG-FA has been widely explored in breast reconstruction, few studies have stratified for the specific type of breast reconstructive procedure. Munabi et al. (18) found the sensitivity and specificity to increase after stratification of reconstruction type. The highest sensitivity and specificity were observed in the patient group undergoing reconstruction with free DIEP and pedicled TRAM flaps, suggesting that ICG-FA may be more reliable during autologous breast reconstruction compared with implant-based. This may be explained by the differences between the flap types in terms of vascular anatomy, flow characteristics, and the contribution from the different vascular plexuses and fascia to the perfusion of the flap.
Autologous reconstruction
Free perforator flaps, as well as pedicled perforator flaps, are supplied by the chosen perforator branching off to supply its territory (Figure 4). The vascular network is supplied by collaterals, also known as direct linking vessels, situated within the subcutaneous tissue, establishing basis for the perforasome/angiosome concept (58,59). These vessels play a critical role in ensuring sufficient perfusion of adjacent skin territories, with a preference for filling perforators originating from the same source artery, as dictated by the third principle of perforators (61). When tissue is transferred, the contribution from collaterals outside the clinical skin territory of the source artery diminishes, potentially resulting in acute ischemia in the periphery of the flap, if the flap design is not thoroughly planned. Pre-operatively, duplex sonography and computed tomography (CT)-angiography are utilised to identify and verify a proper perforator but are insufficient to guide during delineation of the supplied territory. The incorporation of ICG-FA in free flap surgery offers several advantages, including pre-operative localization and delineation of perforators, angiosomes (62) or perforasomes (61), which can guide during flap design, post-operative assessment of flap perfusion, and the opportunity to objectively track the post-operative adaptation of the flap (Figure 5). However, studies exploring objective cut-off values in these contexts are currently lacking.
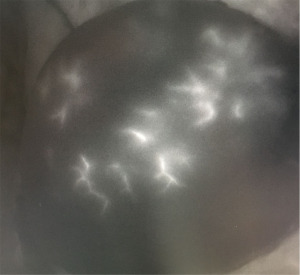
Mastectomy skin flaps
The vascular supply of the breast is presented in Figure 6. During the initial dissection of the mastectomy skin flap, the transection of sympathetic nerves results in the local release of norepinephrine, potentially inducing vasoconstriction persisting for up to 30 hours (63). This phenomenon may manifest as prolonged time-related parameters, diminished intensity-dependent, and diminished combined parameters, potentially contributing to the formerly mentioned overestimation of necrosis rates. However, if the subdermal plexus and ideally the subcutaneous plexuses are preserved, this ensures the continuity of choke vessels. These vessels function as perfusion reserves that can be mobilised if needed, as part of the delay phenomenon (60). ICG-FA potentially offers an opportunity of monitoring the adaption of choke vessels during the early phase and the neovascularization during the late phase of the vascular delay in a post-operative bedside setting (Figure 7). Consequently, progressive shortening of time-related parameters, increasing intensity-dependent parameters, and augmentation of combined parameters may signify improvement of mastectomy skin flap perfusion.
There is a lack of documentation regarding post-operative hemodynamic changes of mastectomy skin flaps in follow-up studies, which could provide an alternative method for predicting mastectomy skin flap viability.
Recommendations for application of ICG-FA and future perspectives
Studies investigating perfusion quantification with ICG-FA in breast reconstruction show a high degree of heterogeneity, demonstrating the need for a consensus regarding an optimal perfusion quantification protocol. Therefore, we present the following suggestions regarding the challenges that quantitative ICG-FA faces before a standardized and optimized protocol can be established.
Parameter selection
Our study emphasizes the nuanced differences across various camera systems, dosing regimens, surgical contexts, and other relevant factors. Our aim was to provide a comprehensive analysis that underscores the importance of considering these factors in predicting mastectomy flap viability based on ICG-FA. The optimal parameter should be reliable, independent of external factors, transferrable between technical setups and patients, and easily accessible. Intensity-dependent parameters have performed inconsistently. Reproducing identical conditions between surgeries is a critical factor that must be managed to extract reliable intensity-dependent parameters. Even, if possible, inter-individual variance is likely to compromise their reliability. However, if intensity-dependent parameters are applied, investigators should describe and provide the quantitative properties of the camera system. Commercially available phantoms can be used to describe the optical properties, depth sensitivity, dynamic range, field homogeneity, and spatial resolutions of the imaging system (64).
Time-related parameters are less susceptible to bias, and ensuring identical camera systems, working distances, and dosages are of less importance when compared with intensity-dependent parameters. Nonetheless, the potential of time-related parameters needs further validation. Only one study has proposed specific cut-off values based on this parameter group (45). In that study, T1 was superior to absolute and relative parameters. Another study observed a significant difference between viable and necrotic tissue for Tmax and T1/2max (19). Furthermore, inconsistencies persist regarding the inclusion of the time interval corresponding to T0 in Tmax and T1/2max. Standardization of the parameter definitions is a critical factor in ensuring comparable results across studies.
For combined parameters, the best performance was observed for slope at T1/2max and slope at Tmax. When cut-off values of 0.4 and 0.2 were applied, these parameters appeared superior in predicting flap outcome compared with relative values based on intensity (19). Combined values can be modified to be based on relative changes in fluorescence intensity by utilisation of normalization, reducing impact from conditional factors. Thus, cut-off values should theoretically be transferrable between set-ups and patients, and further studies are needed for validation.
Hence, we recommend applying the 33% cut-off value based on time-defined intensity-dependent parameters in daily care. However, combined and time-related parameters may be more reliable and reproducible. Therefore, further studies correlating combined or time-related parameters with clinical outcomes are needed to establish objective cut-off values. If possible, we strongly encourage reporting of flap failure reduction rate and revision success rate as these are simple tools for a comprehensive and comparative description of the quality of monitoring devices, allowing to compare results across studies (65).
Interpretation
In a clinical setting, it is more feasible to interpret a perfusion index rather than aiming for a specific value. Hence, objective reference frames for the most frequent procedures must be established, as the methodology will remain subjective until an evidence-based value has proven its reliability. Besides, relative parameters are less susceptible to the impact of inter-individual variation, which is otherwise difficult to standardize. Accordingly, relative values based on absolute intensity have been extensively investigated in breast reconstruction and are currently the most well-documented analysis, but the inability of transferring relative cut-off values between camera and software systems was recently confirmed by our research group (47). Therefore, further studies are needed to confirm that relative indexes not transferrable between intensity-dependent, time-related, and combined parameters.
Pre-investigational surface warming
Multiple studies have stated that ICG-FA overestimates necrosis rate due to low specificity (17,33). However, it has been shown that the specificity can be elevated by performing ICG-FA after surface-warming, decreasing the risk of over-resecting the flap (48,66). Muntean et al. (66) proved that local flap warming at 42 °C allows a more realistic analysis of perfusion. This might have challenged the reliability of the intra-operative measurements performed soon after harvesting the flap, as ICG-FA will exaggerate the areas of hypoperfusion in case of hypothermia due to physiological vasoconstriction. In contrast, heat increases the quality and quantity of vascular perfusion by dilating and opening choke vessels, mimicking the microcirculatory environment encountered at 24 h. Therefore, it has been proposed apply surface warming and use a ≤40% relative cut-off value based on intensity (48).
Dosing regimen
Generally, the luminescence exhibited by a fluorescent material is dependent on the solute concentration, which is affected by the amount of fluid flushing, dose, and possibly site of ICG injection. As the solute concentration increases at lower concentrations, there is a proportional increase in luminescence intensity. However, when the solute concentration continues to increase, the luminescence eventually attains a peak value, followed by a decline in luminescence, commonly referred to as the “quenching phenomenon” (44). It is therefore noteworthy that a highly concentrated solution, despite yielding promising outcomes, may induce a decrease in luminescence. Accordingly, numerous facilities have adopted a concentration of 2.5 mg/mL. Furthermore, if the dosage of ICG remains uniform across all patients, it is susceptible to differences in circulating plasma levels owing to its rapid binding to plasma proteins. However, these aspects are of less importance when using relative values.
Micro-dosing
Another approach to enhancing the reliability and utility is micro-dosing, which has recently gained interest, offering the possibility of performing repeated, short-interval flap perfusion assessment. Furthermore, micro-dosing allows for both intra-operative localisation of angiosomes, perforasomes, perforators, perfusion validation, and continuous monitoring post-operatively without exceeding the maximum recommended daily dose of 2 mg/kg/day. Post-operative measurements may provide benefits compared with intra-operative measurements due to the physiological responses present immediately after surgery, since influence from vasoconstrictors diminish post-operatively, and it is possible to monitor the adaption of the flap.
Hitier et al. (34) evaluated the feasibility of repeated micro-dosing in free flaps. Twenty patients undergoing microsurgical reconstruction were monitored by quantitative ICG-FI intra-operatively and every 6 h for 4 days post-operatively, beginning 2 h after the end of surgery with eighteen injections of 0.025 mg/kg ICG. The first measurement was performed intra-operatively immediately after flap anastomosis to the recipient vessel, adding approximately 5 min to surgery time. Notably, this study utilized a handheld imaging system, facilitating post-operative bedside applications. No adverse effect was observed despite iterative injections. The study showed that repeated dosing of ICG is safe and feasible and may refine post-operative monitoring of free flaps. Fmax predicted vascular complications intra-operatively, while slope and ingress were successfully used for post-operative perfusion assessment.
This innovative approach warrants further investigation. Perfusion Tech has recently devised a novel quantification software, PerfusionWorks, based on repeated micro-dose regimen. Our research group is currently exploring the opportunities in post-operative monitoring with this newly developed concept.
Conclusions
Quantitative software enables the extraction of numerical values that can be used as cut-off thresholds. Intensity-dependent parameters are well-described but susceptible to several variables (ambient light, ICG dose, camera system, working distance, patient-related factors) which challenge their usability. Combined and time-related parameters have shown promising results and are more resilient to inevitable circumstantial variation. They should be preferred once objective cut-off values have been validated. The potential of post-operative flap monitoring based on micro-dosing awaits further validation.
Acknowledgments
Funding: None.
Footnote
Reporting Checklist: The authors have completed the Narrative Review reporting checklist. Available at https://abs.amegroups.com/article/view/10.21037/abs-24-15/rc
Peer Review File: Available at https://abs.amegroups.com/article/view/10.21037/abs-24-15/prf
Conflicts of Interest: All authors have completed the ICMJE uniform disclosure form (available at https://abs.amegroups.com/article/view/10.21037/abs-24-15/coif). T.E.D. serves as an unpaid editorial board member of Annals of Breast Surgery from July 2023 to June 2025. F.T.A. receives grant from Eureka, Eurostars call for R&D and innovation projects (No. 2103-0008413); and received fees during a collaboration with Perfusion Tech Aps, which fully covered all expenses associated with a technically instructive meeting in Copenhagen and provided technical equipment for the animal experiments. J.M.H. received grant from Eureka, Eurostars call for R&D and innovation projects (No. 2103-0008413); and have a co-ownership in Perfusion Tech ApS, which fully covered all expenses in a meeting in Copenhagen and provided technical equipment for the animal experiments. The authors have no other conflicts of interest to declare.
Ethical Statement: The authors are accountable for all aspects of the work in ensuring that questions related to the accuracy or integrity of any part of the work are appropriately investigated and resolved.
Open Access Statement: This is an Open Access article distributed in accordance with the Creative Commons Attribution-NonCommercial-NoDerivs 4.0 International License (CC BY-NC-ND 4.0), which permits the non-commercial replication and distribution of the article with the strict proviso that no changes or edits are made and the original work is properly cited (including links to both the formal publication through the relevant DOI and the license). See: https://creativecommons.org/licenses/by-nc-nd/4.0/.
References
- Koonce SL, Sarik JR, Forleiter CM, et al. A classification system and treatment algorithm for mastectomy flap ischemia in alloplastic breast reconstruction. J Plast Reconstr Aesthet Surg 2020;73:1854-61. [Crossref] [PubMed]
- Khavanin N, Qiu C, Darrach H, et al. Intraoperative Perfusion Assessment in Mastectomy Skin Flaps: How Close are We to Preventing Complications? J Reconstr Microsurg 2019;35:471-8. [Crossref] [PubMed]
- Jallali N, Ridha H, Butler PE. Postoperative monitoring of free flaps in UK plastic surgery units. Microsurgery 2005;25:469-72. [Crossref] [PubMed]
- Davies K, Allan L, Roblin P, et al. Factors affecting post-operative complications following skin sparing mastectomy with immediate breast reconstruction. Breast 2011;20:21-5. [Crossref] [PubMed]
- Meretoja TJ, Rasia S, von Smitten KA, et al. Late results of skin-sparing mastectomy followed by immediate breast reconstruction. Br J Surg 2007;94:1220-5. [Crossref] [PubMed]
- Komorowska-Timek E, Gurtner GC. Intraoperative perfusion mapping with laser-assisted indocyanine green imaging can predict and prevent complications in immediate breast reconstruction. Plast Reconstr Surg 2010;125:1065-73. [Crossref] [PubMed]
- Moyer HR, Losken A. Predicting mastectomy skin flap necrosis with indocyanine green angiography: the gray area defined. Plast Reconstr Surg 2012;129:1043-8. [Crossref] [PubMed]
- Disa JJ, Cordeiro PG, Hidalgo DA. Efficacy of conventional monitoring techniques in free tissue transfer: an 11-year experience in 750 consecutive cases. Plast Reconstr Surg 1999;104:97-101. [Crossref] [PubMed]
- Bui DT, Cordeiro PG, Hu QY, et al. Free flap reexploration: indications, treatment, and outcomes in 1193 free flaps. Plast Reconstr Surg 2007;119:2092-100. [Crossref] [PubMed]
- Chen KT, Mardini S, Chuang DC, et al. Timing of presentation of the first signs of vascular compromise dictates the salvage outcome of free flap transfers. Plast Reconstr Surg 2007;120:187-95. [Crossref] [PubMed]
- Still J, Law E, Dawson J, et al. Evaluation of the circulation of reconstructive flaps using laser-induced fluorescence of indocyanine green. Ann Plast Surg 1999;42:266-74. [Crossref] [PubMed]
- Goncalves LN, van den Hoven P, van Schaik J, et al. Perfusion Parameters in Near-Infrared Fluorescence Imaging with Indocyanine Green: A Systematic Review of the Literature. Life (Basel) 2021;11:433. [Crossref] [PubMed]
- Malagón-López P, Vilà J, Carrasco-López C, et al. Intraoperative Indocyanine Green Angiography for Fat Necrosis Reduction in the Deep Inferior Epigastric Perforator (DIEP) Flap. Aesthet Surg J 2019;39:NP45-54. [Crossref] [PubMed]
- Hembd AS, Yan J, Zhu H, et al. Intraoperative Assessment of DIEP Flap Breast Reconstruction Using Indocyanine Green Angiography: Reduction of Fat Necrosis, Resection Volumes, and Postoperative Surveillance. Plast Reconstr Surg 2020;146:1e-10e. [Crossref] [PubMed]
- Michi M, Verduijn PS, Corion LUM, et al. Assessment of deep inferior epigastric perforator flap perfusion with near-infrared fluorescence: A pilot study and description of a standardized working protocol. J Plast Reconstr Aesthet Surg 2022;75:1171-8. [Crossref] [PubMed]
- Newman MI, Jack MC, Samson MC. SPY-Q analysis toolkit values potentially predict mastectomy flap necrosis. Ann Plast Surg 2013;70:595-8. [Crossref] [PubMed]
- Phillips BT, Lanier ST, Conkling N, et al. Intraoperative perfusion techniques can accurately predict mastectomy skin flap necrosis in breast reconstruction: results of a prospective trial. Plast Reconstr Surg 2012;129:778e-88e. [Crossref] [PubMed]
- Munabi NC, Olorunnipa OB, Goltsman D, et al. The ability of intra-operative perfusion mapping with laser-assisted indocyanine green angiography to predict mastectomy flap necrosis in breast reconstruction: a prospective trial. J Plast Reconstr Aesthet Surg 2014;67:449-55. [Crossref] [PubMed]
- Gorai K, Inoue K, Saegusa N, et al. Prediction of Skin Necrosis after Mastectomy for Breast Cancer Using Indocyanine Green Angiography Imaging. Plast Reconstr Surg Glob Open 2017;5:e1321. [Crossref] [PubMed]
- Van Den Hoven P, Verduijn PS, Van Capelle L, et al. Quantification of near-infrared fluorescence imaging with indocyanine green in free flap breast reconstruction. J Plast Reconstr Aesthet Surg 2022;75:1820-5. [Crossref] [PubMed]
- Sullivan D, Chung KC, Eaves FF 3rd, et al. The level of evidence pyramid: indicating levels of evidence in Plastic and Reconstructive Surgery articles. Plast Reconstr Surg 2011;128:311-4. [Crossref] [PubMed]
- Pogue BW, Rosenthal EL. Review of successful pathways for regulatory approvals in open-field fluorescence-guided surgery. J Biomed Opt 2021;26:030901. [Crossref] [PubMed]
- Hackethal A, Hirschburger M, Eicker SO, et al. Role of Indocyanine Green in Fluorescence Imaging with Near-Infrared Light to Identify Sentinel Lymph Nodes, Lymphatic Vessels and Pathways Prior to Surgery - A Critical Evaluation of Options. Geburtshilfe Frauenheilkd 2018;78:54-62. [Crossref] [PubMed]
- Schouw HM, Huisman LA, Janssen YF, et al. Targeted optical fluorescence imaging: a meta-narrative review and future perspectives. Eur J Nucl Med Mol Imaging 2021;48:4272-92. [Crossref] [PubMed]
- Cha HG. Utility of Indocyanine Green Fluorescence Imaging in Wound Assessment. J Wound Manag Res 2023;19:1-7. [Crossref]
- Holm C, Dornseifer U, Sturtz G, et al. The intrinsic transit time of free microvascular flaps: clinical and prognostic implications. Microsurgery 2010;30:91-6. [Crossref] [PubMed]
- De Lorenzi F, Yamaguchi S, Petit JY, et al. Evaluation of skin perfusion after nipple-sparing mastectomy by indocyanine green dye. Preliminary results. J Exp Clin Cancer Res 2005;24:347-54. [PubMed]
- Newman MI, Samson MC, Tamburrino JF, et al. Intraoperative laser-assisted indocyanine green angiography for the evaluation of mastectomy flaps in immediate breast reconstruction. J Reconstr Microsurg 2010;26:487-92. [Crossref] [PubMed]
- Sood M, Glat P. Potential of the SPY intraoperative perfusion assessment system to reduce ischemic complications in immediate postmastectomy breast reconstruction. Ann Surg Innov Res 2013;7:9. [Crossref] [PubMed]
- Wapnir I, Dua M, Kieryn A, et al. Intraoperative imaging of nipple perfusion patterns and ischemic complications in nipple-sparing mastectomies. Ann Surg Oncol 2014;21:100-6. [Crossref] [PubMed]
- Phillips BT, Fourman MS, Rivara A, et al. Comparing quantitative values of two generations of laser-assisted indocyanine green dye angiography systems: can we predict necrosis? Eplasty 2014;14:e44. [PubMed]
- Ludolph I, Arkudas A, Schmitz M, et al. Cracking the perfusion code?: Laser-assisted Indocyanine Green angiography and combined laser Doppler spectrophotometry for intraoperative evaluation of tissue perfusion in autologous breast reconstruction with DIEP or ms-TRAM flaps. J Plast Reconstr Aesthet Surg 2016;69:1382-8. [Crossref] [PubMed]
- Mattison GL, Lewis PG, Gupta SC, et al. SPY Imaging Use in Postmastectomy Breast Reconstruction Patients: Preventative or Overly Conservative? Plast Reconstr Surg 2016;138:15e-21e. [Crossref] [PubMed]
- Hitier M, Cracowski JL, Hamou C, et al. Indocyanine green fluorescence angiography for free flap monitoring: A pilot study. J Craniomaxillofac Surg 2016;44:1833-41. [Crossref] [PubMed]
- Alstrup T, Christensen BO, Damsgaard TE. ICG angiography in immediate and delayed autologous breast reconstructions: peroperative evaluation and postoperative outcomes. J Plast Surg Hand Surg 2018;52:307-11. [Crossref] [PubMed]
- Hammer-Hansen N, Juhl AA, Damsgaard TE. Laser-assisted indocyanine green angiography in implant-based immediate breast reconstruction: a retrospective study. J Plast Surg Hand Surg 2018;52:158-62. [Crossref] [PubMed]
- Mirhaidari SJ, Beddell GM, Orlando MV, et al. A Prospective Study of Immediate Breast Reconstruction with Laser-Assisted Indocyanine Green Angiography. Plast Reconstr Surg Glob Open 2018;6:e1774. [Crossref] [PubMed]
- de Vita R, Buccheri EM. Nipple sparing mastectomy and direct to implant breast reconstruction, validation of the safe procedure through the use of laser assisted indocyanine green fluorescent angiography. Gland Surg 2018;7:258-66. [Crossref] [PubMed]
- Yang CE, Chung SW, Lee DW, et al. Evaluation of the Relationship Between Flap Tension and Tissue Perfusion in Implant-Based Breast Reconstruction Using Laser-Assisted Indocyanine Green Angiography. Ann Surg Oncol 2018;25:2235-40. [Crossref] [PubMed]
- Wang CY, Wang CH, Tzeng YS, et al. Intraoperative Assessment of the Relationship Between Nipple Circulation and Incision Site in Nipple-Sparing Mastectomy With Implant Breast Reconstruction Using the SPY Imaging System. Ann Plast Surg 2018;80:S59-65. [Crossref] [PubMed]
- Girard N, Delomenie M, Malhaire C, et al. Innovative DIEP flap perfusion evaluation tool: Qualitative and quantitative analysis of indocyanine green-based fluorescence angiography with the SPY-Q proprietary software. PLoS One 2019;14:e0217698. [Crossref] [PubMed]
- Kim J, Kim IK, Jin US, et al. Quantitative assessment of nipple perfusion with laser-assisted indocyanine green imaging in nipple-sparing mastectomy with breast reconstruction. Arch Aesthetic Plast Surg 2019;25:1-8. [Crossref]
- Varela R, Casado-Sanchez C, Zarbakhsh S, et al. Outcomes of DIEP Flap and Fluorescent Angiography: A Randomized Controlled Clinical Trial. Plast Reconstr Surg 2020;145:1-10. [Crossref] [PubMed]
- Ogawa A, Nakagawa T, Oda G, et al. Study of the protocol used to evaluate skin-flap perfusion in mastectomy based on the characteristics of indocyanine green. Photodiagnosis Photodyn Ther 2021;35:102401. [Crossref] [PubMed]
- Mastronardi M, Fracon S, Scomersi S, et al. Role of Qualitative and Quantitative Indocyanine Green Angiography to Assess Mastectomy Skin Flaps Perfusion in Nipple/Skin-Sparing and Skin-Reducing Mastectomies with Implant-Based Breast Reconstruction. Breast J 2022;2022:5142100. [Crossref] [PubMed]
- Pruimboom T, Tuinder SMH, Qiu SS, et al. The impact of using the internal mammary artery as a recipient vessel on medial mastectomy skin flap perfusion in autologous breast reconstruction: An observational study using indocyanine green. J Plast Reconstr Aesthet Surg 2023;84:350-6. [Crossref] [PubMed]
- Lauritzen E, Bredgaard R, Bonde C, et al. An observational study comparing the SPY-Elite®vs. the SPY-PHI QP system in breast reconstructive surgery. Ann Breast Surg 2023;7:12. [Crossref]
- Choudhary S, Khanna S, Mantri R, et al. Role of Indocyanine Green Angiography in Free Flap Surgery: A Comparative Outcome Analysis of a Single-Center Large Series of 877 Consecutive Free Flaps. Indian J Plast Surg 2023;56:208-17. [Crossref] [PubMed]
- Rinker B. A Comparison of Methods to Assess Mastectomy Flap Viability in Skin-Sparing Mastectomy and Immediate Reconstruction: A Prospective Cohort Study. Plast Reconstr Surg 2016;137:395-401. [Crossref] [PubMed]
- Oppermann C, Dohrn N, Yikilmaz H, et al. Continuous organ perfusion monitoring using indocyanine green in a piglet model. Surg Endosc 2023;37:1601-10. [Crossref] [PubMed]
- Betz CS, Zhorzel S, Schachenmayr H, et al. Endoscopic measurements of free-flap perfusion in the head and neck region using red-excited Indocyanine Green: preliminary results. J Plast Reconstr Aesthet Surg 2009;62:1602-8. [Crossref] [PubMed]
- Tange FP, Verduijn PS, Sibinga Mulder BG, et al. Near-infrared fluorescence angiography with indocyanine green for perfusion assessment of DIEP and msTRAM flaps: A Dutch multicenter randomized controlled trial. Contemp Clin Trials Commun 2023;33:101128. [Crossref] [PubMed]
- Bigdeli AK, Gazyakan E, Schmidt VJ, et al. Indocyanine Green Fluorescence for Free-Flap Perfusion Imaging Revisited: Advanced Decision Making by Virtual Perfusion Reality in Visionsense Fusion Imaging Angiography. Surg Innov 2016;23:249-60. [Crossref] [PubMed]
- Mayr M, Holm C, Höfter E, et al. Effects of aesthetic abdominoplasty on abdominal wall perfusion: a quantitative evaluation. Plast Reconstr Surg 2004;114:1586-94. [Crossref] [PubMed]
- Miyazaki H, Igari K, Kudo T, et al. Significance of the Lateral Thoracic Artery in Pectoralis Major Musculocutaneous Flap Reconstruction: Quantitative Assessment of Blood Circulation Using Indocyanine Green Angiography. Ann Plast Surg 2017;79:498-504. [Crossref] [PubMed]
- Schöpper S, Smeets R, Gosau M, et al. Intraoperative ICG-based fluorescence-angiography in head and neck reconstruction: Predictive value for impaired perfusion of free flaps. J Craniomaxillofac Surg 2022;50:371-9. [Crossref] [PubMed]
- Ye X, Liu XJ, Ma L, et al. Clinical values of intraoperative indocyanine green fluorescence video angiography with Flow 800 software in cerebrovascular surgery. Chin Med J (Engl) 2013;126:4232-7. [Crossref] [PubMed]
- Mastronardi M, Fracon S, Scomersi S, et al. Preliminary Results From A Prospective Study On Qualitative And Quantitative Indocyanine Green Angiography To Assess Mastectomy Skin Flaps Perfusion. Journal of the American College of Surgeons 2023;236:S7. [Crossref]
- Van Den Hoven P, Osterkamp J, Nerup N, et al. Quantitative perfusion assessment using indocyanine green during surgery - current applications and recommendations for future use. Langenbecks Arch Surg 2023;408:67. [Crossref] [PubMed]
- Lee BT, Matsui A, Hutteman M, et al. Intraoperative near-infrared fluorescence imaging in perforator flap reconstruction: current research and early clinical experience. J Reconstr Microsurg 2010;26:59-65. [Crossref] [PubMed]
- Saint-Cyr M, Wong C, Schaverien M, et al. The perforasome theory: vascular anatomy and clinical implications. Plast Reconstr Surg 2009;124:1529-44. [Crossref] [PubMed]
- Taylor GI, Pan WR. The Angiosome Concept And Tissue Transfer. Dodwell P. editor. St. Louis: Karen Berger; 2014.
- Hamilton K, Wolfswinkel EM, Weathers WM, et al. The Delay Phenomenon: A Compilation of Knowledge across Specialties. Craniomaxillofac Trauma Reconstr 2014;7:112-8. [Crossref] [PubMed]
- Heeman W, Vonk J, Ntziachristos V, et al. A Guideline for Clinicians Performing Clinical Studies with Fluorescence Imaging. J Nucl Med 2022;63:640-5. [Crossref] [PubMed]
- Iblher N, Eisenhardt SU, Penna V, et al. A new evaluation tool for monitoring devices and its application to evaluate the implantable Doppler probe. J Reconstr Microsurg 2010;26:265-70. [Crossref] [PubMed]
- Muntean MV, Ardelean F, Strilciuc S, et al. Flap warming improves intraoperative indocyanine green angiography (ICGA) assessment of perfusion. An experimental study. J Plast Reconstr Aesthet Surg 2019;72:1150-6. [Crossref] [PubMed]
Cite this article as: Andersen FT, Hasenkam JM, Damsgaard TE. Indocyanine green angiography—current status on quantification of perfusion: a narrative review. Ann Breast Surg 2024;8:33.